In areas such as intravascular ultrasound (IVUS) and intracardiac echocardiography (ICE), the limits of real-time 3D medical ultrasonic imaging are being pushed with the introduction of Piezoelectric Micromachined Ultrasonic Transducers (pMUTs).
Offering higher operating frequencies with a smaller form factor, pMUTs are a viable alternative to traditional lead zirconate titanate (PZT) bulk ceramic transducers. A cost-effective approach for large volume production of high-density 2-D arrays is provided by the fabrication of pMUTs as a microelectromechanical system (MEMS) using well-established semiconductor manufacturing processes. They can also interface directly with on-chip integrated circuits for signal processing. This helps to mitigate some of the challenges faced with catheter-based ultrasonic imaging. For example, the complexity of the interconnect and limitations in terms of performance of bulk PZT transducers.
pMUT Structure and Fabrication
The pMUT structure is made up of a cavity covered by a flexible piezoelectric membrane. This will mechanically deform with applied electrical stimulation. A spin-coating process is used to deposit a PZT film onto a silicon-on-insulator (SOI) substrate with Ti/Pt electrode layers. A two-dimensional array of elements is formed by photolithographically patterning and etching the PZT film and electrode layers. A deep reactive ion etch (DRIE) process is used to etch a cavity in the bulk silicon behind each element.
Figure 1 illustrates the basic structure of a pMUT element.
The desired operation is produced for these pMUT devices, by positioning the cavity, PZT film layer, and electrodes in a specific conformation (Fig. 2). To restrict electrical shorting to the ground electrode plane beneath the PZT, the top electrode layer is patterned smaller than the underlying PZT film. The cavity undercuts the PZT film layer by several micrometers as it is shorter in one dimension than the other. The cavity extends beyond the dimensions of the PZT film in the longer dimension. As a result, there are different boundary conditions in the two lateral dimensions. This promotes vibration of the structure as a plate bounded differently on adjacent sides as opposed to a membrane-bounded equally on each side. The bandwidth of the devices is increased by the rectangular shape and boundary conditions of the pMUTs.
.jpg)
Figure 1: Cross section of a pMUT laminate structure with component layers.
.jpg)
Figure 2: Top-down diagram of the pMUT structure shown with positioning of the cavity, PZT, and electrode. Cited pMUT sizes correspond to electrode length.
Motivation for Optical Measurement of pMUT Vibration
Only experimental measurement of the acoustic and electrical response of pMUT arrays forms our current understanding of their operation.
There has been insufficient theory regarding plate vibration to characterize the relationship between pMUT structure and performance [1-3]. The vibration of the smaller elements was described through modeling the pMUTs as a transverse resonator in the length dimension. However, it was not possible to model the larger elements as a fundamental mode of either a transverse or plate resonator (Fig. 3). There wasn’t a single higher order mode of either model that was sufficient to describe the measured high-frequency operation (4 to 8 MHz measured, <2 MHz expect- ed). However, operation in a complex higher-order plate mode was suggested by observing a thickness-dependence in operating frequency [1]. It was realized that, in high-frequency vibration, confirmation of fundamental mode operation for smaller elements and complex higher-order mode vibration of the larger elements could be reached through direct optical measurement of the pMUT elements.
.jpg)
Figure 3: Measured frequency versus etched length for elements of different thickness (dashed lines) plotted with theoretical calculations for (a) flextensional plate vibration and (b) transverse resonance.
Vibration Measurement Results
The individual 75 µm and 200 µm pMUT elements in vibration were visualized using the MSA-400 Micro System Analyzer (new version MSA-600 up to 2.5 GHz was introduced in 2019). As shown in Figure 4, the 75 µm elements could operate in a fundamental mode. However, assumptions about the vibrational boundary conditions were found to be potentially incorrect as displacement was observed over a larger area than expected. As demonstrated in Figure 5, the measurement of displacement across both axes of the 75 µm elements uncovered that the dimensions over which the pMUT vibrates might be up to 10% greater than previously anticipated.
The differences observed between a pMUT’s theoretical and measured frequencies of operation may be influenced significantly by this increase motion. Hypotheses of higher-order operation of these larger elements were confirmed through visualization of the 200 µm pMUT elements (Fig. 6). Fundamental mode vibration was observed at frequencies <2 MHz. However, what appeared to be a complex combination of higher-order vibrational modes were revealed with additional peaks at 5.5, 5.7, and 7.8 MHz. This likely contributed to the acoustic output of the larger devices observed in previous measurements [3].
.jpg)
Figure 4: Vibration of a 75 μm pMUT element at 6.75 MHz in a 14x14 2-D pMUT array.
.jpg)
Figure 5: Measurement of displacement along the length and width dimensions of a 75 μm pMUT element.
.jpg)
Figure 6: Vibration of a 200 μm pMUT element at a) 5.5 MHz, b) 5.7 MHz, c) 7.8 MHz showing different deflection (mode) shapes. The fundamental mode at frequencies <2 MHz not shown. Single element in a 5x5 2-D pMUT array.
Conclusions
The characterization of pMUT devices presented unusual and demanding measurement requirements. These included high speed (~10 MHz) and high resolution (<1 µm). They were made possible by the laser vibrometer capabilities of the MSA-400 Micro System Analyzer.
A wealth of information has been revealed about the performance of these pMUT devices in vibration through the use of the MSA-400 laser vibrometer to visualize the surface. The operation of larger elements in a higher order mode and the revelation of uncertain boundary conditions have led to a better understanding of how pMUTs vibrate. This, in turn, will help to advance theoretical models. Further insights into pMUT vibration characteristics may be achieved through future experimentation, which will enable further refinement of their performance as a viable medical ultrasonic transducer.
References
- D. E. Dausch, J. B. Castellucci, D. R. Chou, O. T. von Ramm, “Theory and Operation of 2D Array Piezoelectric Micromachined Ultrasound Transducers,” IEEE Transactions on Ultrasonics, Ferroelectrics and Frequency Control 55(11), 2484-2492 (2008).
- D. E. Dausch, J. B. Castellucci, D. R. Chou, K. H. Gilchrist, O. T. von Ramm, “Performance of Flexure-Mode pMUT 2D Arrays,” Proc. IEEE Ultrasonics Symposium, 1053-1056 (2007).
- D. E. Dausch, J. B. Castellucci, D. R. Chou, O. T. von Ramm, “Piezoelectric Micromachined Ultrasound Transducer (pMUT) Arrays for 3D Imaging Probes,” Proc. IEEE Ultrasonics Symposium, 930-933 (2006).
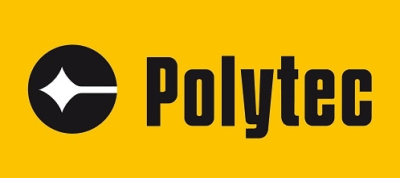
This information has been sourced, reviewed and adapted from materials provided by Polytec.
For more information on this source, please visit Polytec.