To evaluate the performance of MEMS devices and to develop strategies for process improvement, characterization of their motion is now a necessity. MEMS devices now have additional integrated functionality such as the incorporation of thin piezoelectric layers.
This has led to novel devices that require detailed measurement and characterization in operationally representative environmental conditions. Furthermore, changes in residual stresses caused by manufacturing processes can result in reduced performance of the device, which causes further challenges for measurement. As such, a methodology is now required to assess the performance of these functional materials at extremely small sizes.
Introduction
The United Kingdom’s national standards laboratory, the National Physical Laboratory (NPL), is an internationally respected and independent center of excellence for research and development, and knowledge transfer in measurement and materials science. The Functional Materials group at NPL has integrated a Polytec micro-scanning laser Doppler vibrometer with an environmental chamber to characterize MEMS devices at the nano-scale over a wide range of vacuum conditions. To examine the motion of MEMS devices over a wide range of pressures (from 1 bar (atmospheric), down to 10–5 mbar) they developed a specialized laser Doppler vibrometer (LDV).
.jpg)
Figure 1: Photograph of the environmental chamber, showing a sample attached to the vacuum-compatible XY stages. The long-focal length lens can be seen above the sample, which is attached to the Polytec Micro Systems Analyzer Differential Vibrometer.
.jpg)
Figure 2: SEM image of the 4 mm diameter PZT circular membrane. Resonant modes are shown in the image to the right.
Benefits of Polytec’s MSA-400 Micro System Analyzer Combined with a Vacuum Chamber
A Polytec MSA-400- PM2-D Micro System Analyzer (new MSA-600 up to 2.5 GHz now available) is utilized by NPL’s system along with long-distance objective optical lenses. Such lenses can reduce the beam size down to 1 μm. This enables the characterization of samples with lateral sizes of less than10 μm. The Polytec system was chosen for the following reasons:
- Its small spot size and with that an excellent lateral spatial resolution
- Its ability to measure through a vacuum window
- Its differential measurement capability
Broadband stimuli like periodic chirp and white noise can be used to achieve device excitation. Alternatively, for specific frequencies excitation can be achieved using single-frequency waveforms such as sine or square. Velocity or displacement profiles showing the out-of-plane motion with a resolution greater than 10 pm can be produced by scanning the laser across the sample.
Scanning of the sample in the normal operating mode is via the internal optics of the MSA-400. NPL has incorporated a set of X-Y vacuum-compatible stages into the MEMS chamber for larger samples (up to 25 mm). Ordinarily employed for sample positioning and alignment, these stages can also be used to scan the sample and generate the motion profile. The study of devices under more realistic working conditions is realized with the combination of a scanning unit with a devoted, flexible design vacuum chamber.
Sophisticated Data Processing
The velocity signal from the vibrometer controller was imported into a lock-in amplifier (Stanford Research Systems SR830). The reason for this was two-fold. Firstly, velocity/displacement and phase information can be easily extracted. Secondly, far smaller excitation voltages are permitted as the use of lock-in techniques allows for reduced noise levels when obtaining vibrational information at a single frequency. Initially, the position of the resonant peaks of a piezoelectric MEMS device was determined by recording a frequency sweep. The vibration mode was then mapped by either laser or sample scanning.
Example: Piezoelectric (PZT) Transducer Membrane
Tests have been carried out on a 4 mm macro-scale PZT membrane (Fig. 2) and an atomic force microscopy (AFM) tip (Fig. 3) to demonstrate the scale over which the scanning vibrometer system can operate. To compare the effects on their dynamic response measurements were taken in air and in a vacuum.
A sinusoidal voltage was directly applied between top (inner) and bottom electrodes to excite the PZT membrane. This exhibited a fundamental mode of vibration at a frequency of 20.78 kHz in air and 17.81 kHz under a vacuum of 4 x 10–5 mbar (see figure 4). A sealed air gap that exists behind the sample membrane and the mounting substrate causes this reduction in frequency.
On reducing the pressure, an increase in frequency is observed for “freely” vibrating samples due to the reduction in air damping acting on the sample. Further vibrational modes can also be seen in addition to the expected higher-order harmonics. This result would be more difficult to observe with a single-point vibrometer. When compared to physically scanning the sample, laser scanning can save time for smaller devices, and can also reduce the effects of lateral motion and backlash of the scanning stages. A complete scan of the out-of-plane motion can be obtained in several minutes dependent on the spatial resolution and frequency range required.
.jpg)
Figure 3: SEM image of the Veeco UltraleverTM AFM tip. Resonant modes are shown below.
.jpg)
Figure 4: Resonant modes of the PZT membrane obtained under a vacuum of 4 x 10–5 mbar (fundamental, first and second order).
.jpg)
Figure 5: Maximum amplitude resonant modes of the AFM tip obtained under a vacuum of 3 x 10–5 mbar.
Experimental Setup
An oil-free environment for sample examination is provided with the use of a diaphragm-backed turbomolecular pump. A good RFI/EMI shielding with good optical clarity is enabled by directing the LDV laser beam through a specially coated covered glass window.
Computer control of the vacuum stages is allowed for with the standard ports, including in the MEMS vacuum chamber (see Figure 1).
Sample excitation for the dynamic analysis is afforded via electrical feed-throughs. Future upgradeability, including optionality to study sample motion as a function of temperature and humidity, is configured with the sample chamber.
Example 2: AFM Tip
The lateral resolution of the system is demonstrated through the results from the AFM tip (feature size ~ 20 μm) as shown in Figure 3. A V-shaped cantilever was scanned in both air and under vacuum. The resonant frequency of the cantilever was 62.12 kHz in air and 62.59 kHz at a pressure of 3 x 10–5 mbar. As shown in Figure 5, the amplitude of vibration increased by a factor of approximately 50. At reduced operating air pressures, an increase in resonant frequency and enhanced Quality factor was observed. This is because as the cantilever resonates in its local environment, it experiences a reduction in the frictional energy dissipating forces. Tools such as finite element analysis can be subsequently used to compare this data to a software-modeled response. Calculation of the force constant is possible with knowledge of the fundamental frequency of the tip. A comparison can then be made with the values provided by the manufacturers.
Example 3: SNOM Gold Probe
Figure 6a shows data taken from a 250 μm diameter gold probe used for a scanning near-field optical microscope (SNOM) using NPL’s system. An etched gold probe is driven at resonance with amplitude of around 1 nm by a small piezo element. This is required for the SNOM to successfully operate. To reduce the area over which information is obtained, the amplitude is kept small.
Scanning over the length of a relatively long probe (approximately 6 mm in length) showed that the fundamental mode of vibration was, in fact, a higher-order harmonic vibration. If the probe was excited at this higher frequency then this would complicate SNOM operation. Figure 6b shows the frequency response of the apex of a shorter probe (approximately 2 mm in length). It was shown that the probe only required a voltage of around 0.6 mV to produce a tip displacement of 1 nm at a resonant frequency of 22.06 kHz.
.jpg)
Figure 6a: Scanning LDV of the SNOM gold probe.
.jpg)
Figure 6b: Frequency response from 2 mm long Au SNOM tip. The LDV image shows that the fundamental mode is indeed being excited, rather than a higher-order harmonic mode of vibration.
Conclusions and Outlook
This work concentrated on obtaining high-resolution vibrometry scans from various samples of interest. Rapid data acquisition and processing by utilizing FFT analysis are advantages offered by NPL’s Polytec MSA-400-PM2-D (differential) Micro System Analyzer. It also permits in-plane vibrational analysis. A unique characterization system for out-of-plane and in-plane dynamic analysis of MEMS and macro-sized devices is provided through a combination with NPL’s vacuum chamber operating down to 10–5 mbar with optional temperature/humidity control.
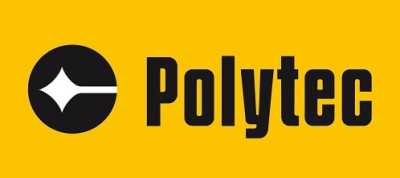
This information has been sourced, reviewed and adapted from materials provided by Polytec.
For more information on this source, please visit Polytec.