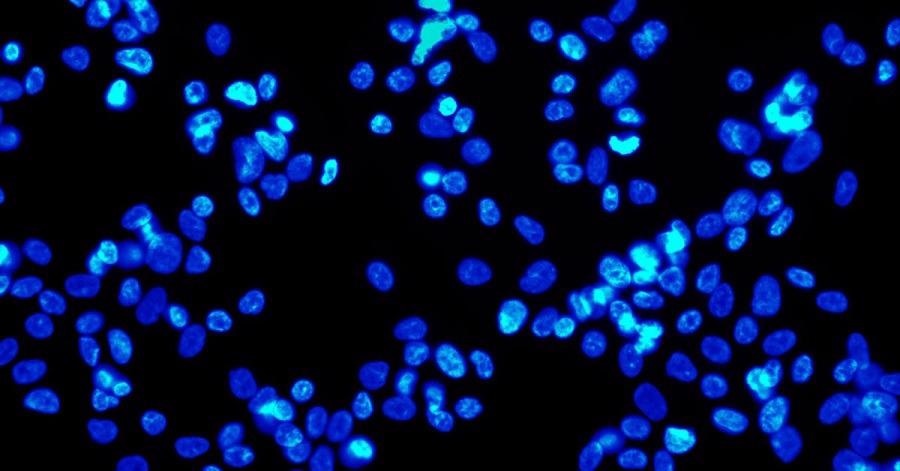
Image Credit: FLIR Systems
Accurate color reproduction, high spatial resolution, and better sensitivity in low light conditions are all usually required in research and diagnostic biomedical applications. In many cases, some combination of all three factors is needed to improve the reliability of data.
Having the correct histology camera, microscopy camera, epifluorescence camera, cytology/cytogenetics camera, etc., is vital for reliable data for research purposes or for giving a proper diagnosis in a clinical application.
In the following article, machine vision cameras for bioscience applications and which is best for a certain application will be explored. This includes a number of aspects to consider when choosing a machine vision camera for life science and biomedical applications.
Application Specific Factors to Consider
Resolution and Color Accuracy
The resolution needed depends on the magnification of the structure of interest in the sample relative to the size of pixels on the camera. For example, high resolution in a microscopy application can be attained using a 2 MP camera, a 25 MP camera, or anything in between.
The magnification of the structure of interest in the sample by the optics relative to the size of the pixels on the camera is key to making this decision. The first step is to determine the size of the smallest structure in the sample that you wish to resolve to choose the best camera options for the desired resolution.
Next, this must be multiplied by the lens magnifications in the optical system. This will give the size of these structures when they are projected on the camera sensor. The camera should be able to resolve the structure if the size of the structure is at least 2.33 (Nyquist) times the size of a pixel on the camera sensor.
For instance, if the size of these projected structures is around ~8 um, then a camera with 3.45 um pixels should be able to resolve those structures. There are some other techniques that can be used to measure resolution (e.g., line pairs) but this is a simple calculation to find suitable camera choices to test.
Imaging applications like cytology, histology, and cytogenetics work with a wide range of white light (between ~400 nm and 700 nm) or utilize a selected wavelength within this range, for example, 565 nm.
If the specimens in these samples are not fixed or living, they can be exposed to bright light levels without the risk of killing or stain fading the sample. The key requirement for the camera under these conditions is color reproduction and high resolution, so low light sensitivity is not a key factor.
Sensitivity, Quantum Efficiency, and Dynamic Range
The challenge for imaging applications with live specimens is to avoid exposing the sample to too much light. This would kill the specimen or bleach fluorescent molecules. Typically, these applications employ a method known as epifluorescence. Epifluorescence methods can be utilized on both live and fixed specimens.
Some specimens are costly or rare, and the process of creating samples can be expensive in labor and materials. So, a system that preserves the quality of the samples can help to decrease the ongoing cost of these imaging applications.
Epifluorescence utilizes a high energy wavelength which is filtered to excite the sample to emit a low energy wavelength and that low energy wavelength is relayed back to the camera.
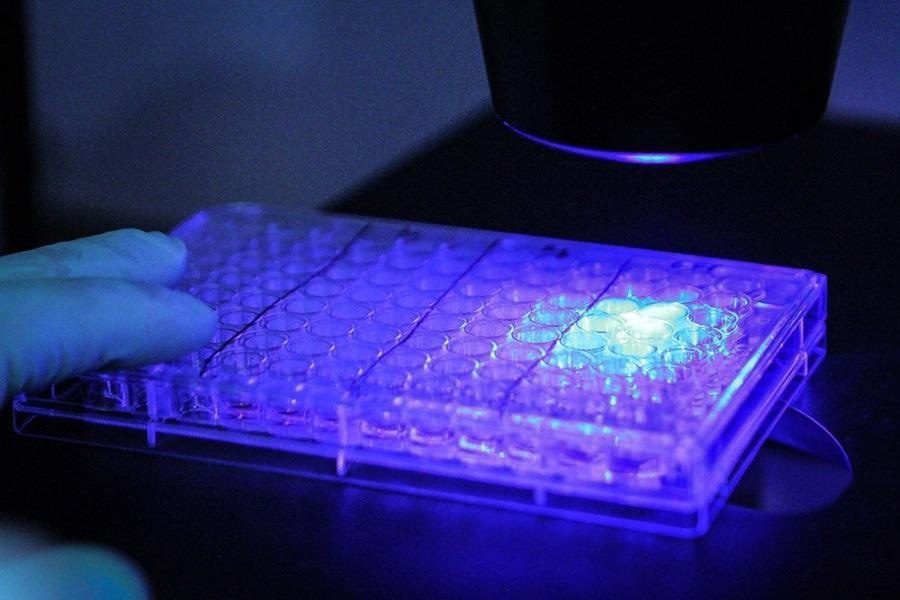
Image Credit: FLIR Systems
The key requirement under these conditions is sensitivity as this enables the utilization of less intense, damaging light on the sample. Even when the emission light is low energy, a camera with excellent sensitivity can supply high quality images.
Quantum efficiency is the percentage of photons converted to electrons at a particular wavelength, and this is where a high number is required. The number of photons required to attain a signal equivalent to the noise observed by the sensor is absolute sensitivity and the lower the number the better.
The ratio of signal to noise, including temporal dark noise (the noise in the sensor when there is no signal), is known as dynamic range; the higher the better.
Compared to color equivalents, monochrome models usually have better performance in low light. Look at the detailed EMVA Imaging Performance document for the model for details of a model’s imaging performance.
Combination of Factors
camera models which offer Sony’s new conversion gain feature should be used for applications which utilize both white light and epifluorescence. This supplies the ability to optimize the sensor for high saturation capacity or high sensitivity.
For low light environments, high conversion gain is ideal because read noise is minimized, yielding a low Absolute Sensitivity threshold which is perfect for detecting weak signals with short exposures.
As saturation capacity is maximized, supplying better dynamic range, low conversion gain is ideal for brightly lit conditions. The 12-bit ADC will limit the maximum dynamic range,
Choosing the Right Cameras
When selecting a camera, a newer CMOS sensor is always a good place to begin. Newer sensors usually provide better performance and may be lower in price.
Additionally, if the application in question requires the purchase of many cameras over a number of years (for example, the ongoing manufacture of a diagnostic instrument), then it is vital to choose a camera that is not at the end of its life cycle or the user would have to incur the cost of designing-in a replacement camera prematurely.
FLIR manufactures more than 200 variants of machine vision cameras that fit broadly into three camera families which utilize the latest CMOS sensors: Oryx, Blackfly S, and Firefly.
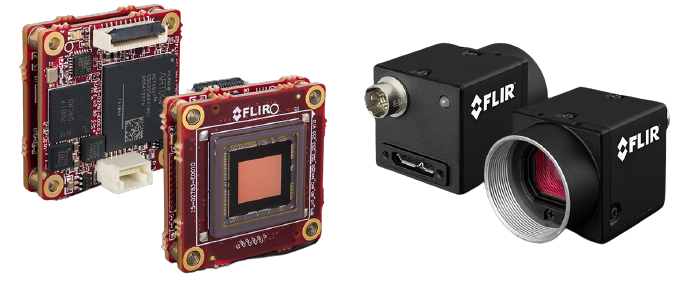
Image Credit: FLIR Systems
The Blackfly S camera family provides the widest range of form factors, sensors, and interfaces. The cameras with every model provided in both USB3 and GigE variants are extremely versatile and simple to incorporate during the design-in phase.
Board level Blackfly S versions are miniaturized versions of the full featured cased variety from FLIR and are especially suited to embedded and space constrained applications. The excellent price-to-performance ratios, a wide variety of features, and resolutions of up to 24 MP make them an ideal choice for life science and biomedical applications.
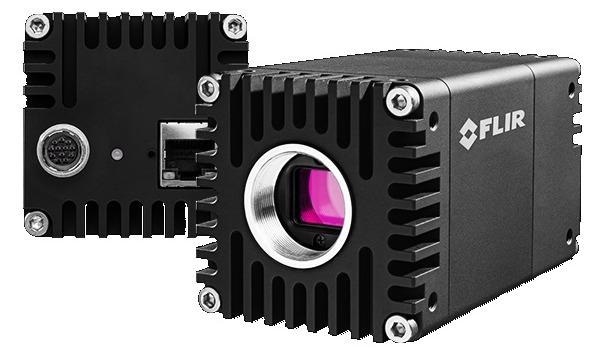
Image Credit: FLIR Systems
The Firefly camera family provides a lightweight, very small case form factor, low price and low power. The Firefly DL model can also run a previously trained neural network which can be utilized for object classification or detection.
The Oryx camera family provides high resolution sensors paired with the quick 10 GigE interface, permitting the capture of 4K resolution, 12-bit images at over 60 FPS. Oryx’s 10 GBASE-T interface is a widely deployed, proven standard which supplies reliable image transfer at cable lengths over 50 meters on inexpensive CAT6A or more than 30 m on CAT5e.
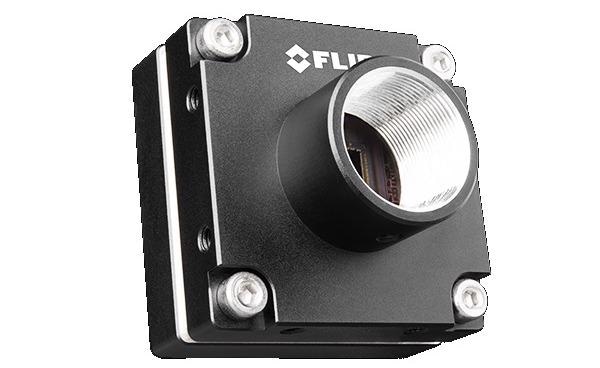
Image Credit: FLIR Systems
All FLIR machine vision color cameras have the ability to customize color reproduction in the form of different white balancing options and the utilization of a unique color correction matrix. These features are crucial in biomedical imaging, where accuracy of colors can mean different things depending on visual human analysis for diagnosis vs. a machine readable format for data accuracy.
The FLIR machine vision Blackfly S, Oryx, and Firefly camera families can also be controlled and programmed by utilizing GenICam3 and the Spinnaker SDK which has been designed ground-up with ease of development and deployment in mind, enabling quicker application development and testing.
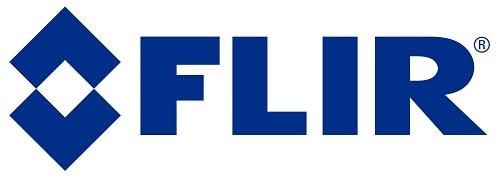
This information has been sourced, reviewed and adapted from materials provided by FLIR Systems.
For more information on this source, please visit FLIR Systems.