Sep 29 2014
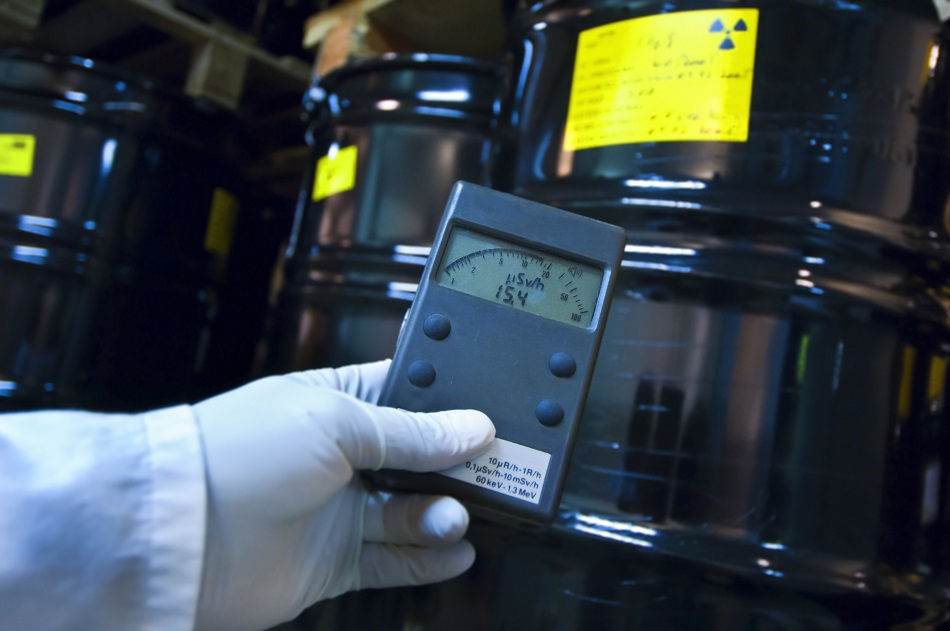
Image Credit: zlikovec/Shutterstock.com
A scintillation counter is a device used for detecting and measuring ionizing radiation. It includes a scintillator that generates light photons in response to incident radiation, which is a sensitive photomultiplier tube that converts light to an electrical signal and also includes the needed electronics to process the photomultiplier tube output.
Scintillation counters are used generally because they can be made at a low cost, but also offer excellent quantum efficiency and can determine the energy of incident radiation and the intensity.
How Does it Work?
When the scintillator is struck by a charged particle, the atoms are excited and photons are emitted. These are directed at the photocathode of the photomultiplier tube, which emits electrons by the photoelectric effect. Electrostatic acceleration of these electrons takes place and an electric potential causes them to strike the first dynode of the tube. The impact of one electron on the dynode releases several secondary electrons that are accelerated to strike the second dynode. The impact of each successive dynode releases electrons so that there is an amplifying effect at each dynode stage. The potential of each stage is higher than the previous one so that an accelerating field is formed.
The resulting output signal at the anode is in the form of a measurable pulse for each photon that is detected at the photocathode and passed to the processing electronics. The pulse holds data about the energy of the initial incident energy on the scintillator. This allows the user to measure energy and intensity of the radiation.
Research
In 2012, the University of Florida researchers discovered a new optically transparent material based on specially designed nanoparticles that when integrated in scintillation counters allow economical sensitive ionizing radiation. This technique is not just highly effective for radiation detection, it is also versatile and can be suited to a number of different radioactive conditions and different types of radiation. Furthermore, production is more economical than conventional single crystal growth manufacturing methods.
Professor Hiroki Kanda delivered a presentation in 2012 on the development of a scintillation counter with multi-pixel photon counters (MPPC) readout for the internal tagging system. The team worked on the development of an electron counter array for the photon tagging spectrometer at the Research Centre for Electron Photon Science, Tohoku University (ELPH). The MPPC from Hamamatsu were selected as photon sensors for the scintillation counters. The counter is rectangular prism-shaped with a square cross section of 3 mm combined with the MPPC. A prototype of the electron detector was fabricated and a beam test was performed.
Applications
Scintillation counters are used for the following applications:
- Pharmaceutical, academic research
- Nuclear power and environmental applications
- Radioactive contamination
- Cellular research, epigenetics and cancer research
- In vivo and ELISA alternative technologies
- Protein interaction and detection
- Screening technologies
Furture Developments
It is anticipated that in the future these devices will have higher speed, better sensitivity and lower noise. Further improvement will include the integration of a pre-amp within the device as part of its fundamental structure. An advantage of these detectors is the ability to fabricate them into odd shapes and sizes and tune them for spectral sensitivity and they may be quite economical.
In the coming years, the small size and cost of computers along with their number crunching capability will create excellent scintillation measurement systems. For liquid scintillation counting it is expected that these computers will be combined with artificial intelligence. The instrument of the future will initiate the measurement cycle by taking a background and processing the data in several ways.
While measuring each sample, the response spectrum will be studied by pattern recognition techniques to identify the isotopes present, the impact of both fluorescence and color quenching, sample changes during counting, volume corrections and chemiluminescence may be indicated. The true disintegration rate of each nuclide present will be determined along with the probable measurement error. The instruments of the future will be self-learning and may learn to re-program itself.
Geiger Counter
A Geiger counter or a Geiger-Muller tube is used for detecting and measuring all kinds of radiation including alpha, beta and gamma. It includes a pair of electrodes enclosed by a gas (normally argon or helium) and a high voltage exists between the electrodes. Radiation entering the tube ionizes the gas and electrons and ions are attracted to the electrodes, resulting in an electric current being produced. The current pulses are counted by a scaler and a count is obtained whenever the gas is ionized by radiation.
The device includes a tube, counter and power supply. The tube is normally cylindrical with a wire across the center and the counter and power supply have timer options and voltage controls.
Three types of Geiger counters are being currently used:
- Gamma-ray counters for gamma rays, cosmic rays and x-ray measurement
- Photoelectric quantum computers that combine features of counters and photo-cells and are used for the measurement of weak ultraviolet light
- Particle counters for measurement of alpha, beta, and H particles
Research
In 1908, at the University of Manchester, Hans Geiger along and Ernest Rutherford engineered a technique to detect alpha particles that were later used in the Geiger Muller tube. This counter could detect only alpha particles and was part of a larger experimental device. The basic ionization mechanism discovered between 1897 and 1901 was termed as the Townsend discharge after its inventor John Sealy Townsend. In 1928, Geiger and Walther Muller designed the sealed Geiger tube that was capable of sensing more types of ionizing radiation and began to be used as a practical radiation sensor.
Muting Reinvented - Smart Process Gating
Modern Geiger counter versions make use of the halogen tube discovered by Sidney H. Liebson in 1947. This was an advancement of the earlier Geiger tube and was advantageous due to its low operating voltage and long life.
N. Veall from the British Institute of Radiology designed a Geiger Muller counter in 1948 of the jacketed tube type to measure beta rays and also designed a convenient way of mounting in a lead shield. The obtained counting rate when the counter is filled is proportional to the amount of radioactive substance in the solution.
Techniques for applying error correction because of dead time of the counter and for changes in specific gravity of the solution are provided along with a calibration curve showing the counter efficiency for a number of radioactive isotopes. This counter was used for measuring radioactivity of plasma, blood and urine in medical tracer experiments.
Applications
The Geiger Muller counter is used for a number of applications based on its design:
- Particle detection - The counter was initially used to detect alpha and beta particles and the instrument is still used for this purpose. For low energy beta particles and alpha particles, the end window type of the GM tube is used since these particles have a restricted range even in free air and are stopped by a solid material. The design of the end window is such that it is thin enough to enable particles to pass through with the least attenuation and the normal window density is 1.5 - 2.0 mg/cm2. However, a Geiger counter with an end window probe is not capable of differentiating between alpha and beta particles. The pancake Geige Muller detector is a modification of the end window probe type and is typically used as a contamination monitor for alpha/beta rays. A thin-walled windowless tube is also capable of detecting high-energy beta particles.
- Gamma and X-ray detection - It is possible to use Geiger counters for the detection of gamma radiation where the windowless tube is used. Efficiency is just 1% because of low gamma interaction with the tube. High energy gamma radiation depends on the interaction of photon radiation with the material in the tube wall - normally 1 – 2 mm of chrome steel for producing electrons that can enter and ionize the fill gas. This is essential since there is a low gas density in the tube and most high energy gamma photons pass through without being detected. For low energy photons, another technique is used involving direct interaction with gas in a long thin walled tube. This design offers an added gas volume and a higher chance of particle interaction. However this still allows low energy photons to enter the gas through the thin wall.
- Neutron detection - A Geiger tube modification is used for neutron measurement using Helium 3 or boron trifluoride. A plastic moderator is used for slowing down of neutrons, and an alpha particle is created in the detector, making neutron counting possible. The Geiger principle is also used in area gamma alarms used for the protection of personnel and in interlock and process measurement applications. A Geiger tube acts as the sensor but the processing electronics will be more advanced and reliable when compared to a hand-held survey meter.
Furture Developments
Further developments in Geiger counters include advanced features and it is believed that this trend will continue for more sophisticated devices.
Gammapilot FTG20 is a sophisticated instrument for point-level detection from Endress+Hauser and features excellent quality, high safety for users and processes and high cost efficiency across the complete life cycle. Gammapilot FTG20 is based on Geiger-Muller technology and has the highest measurement sensitivity for the automation industry. This device finds applications in petrochemical, chemical, oil and gas, paper and primary industries. It can operate at ambient temperatures of -40 to +120°C thus enabling global usage irrespective of climatic zones.
Another development found in the Geiger counter is the Muller 'Guardian Ray'. It is fully designed and manufactured in Italy. Guardian Ray is not just a sensitive Geiger counter but is also an effective dosimeter digital data logger which has a considerable internal memory from a megabit. It permits the storage of measurements performed in time intervals that can be configured by the user then the same can be stored in a PC, so that real time data can be downloaded to your computer.
Sources and Further Reading
This article was updated on 17th February, 2020.