Apr 7 2021
Alongside rising global population levels is the continual development and expansion of various industries, including pharmaceuticals, refining and manufacturing. In spite of their differences, each of these industries is responsible for a generation of extremely contaminated waters and has a vested interest in ensuring water quality.
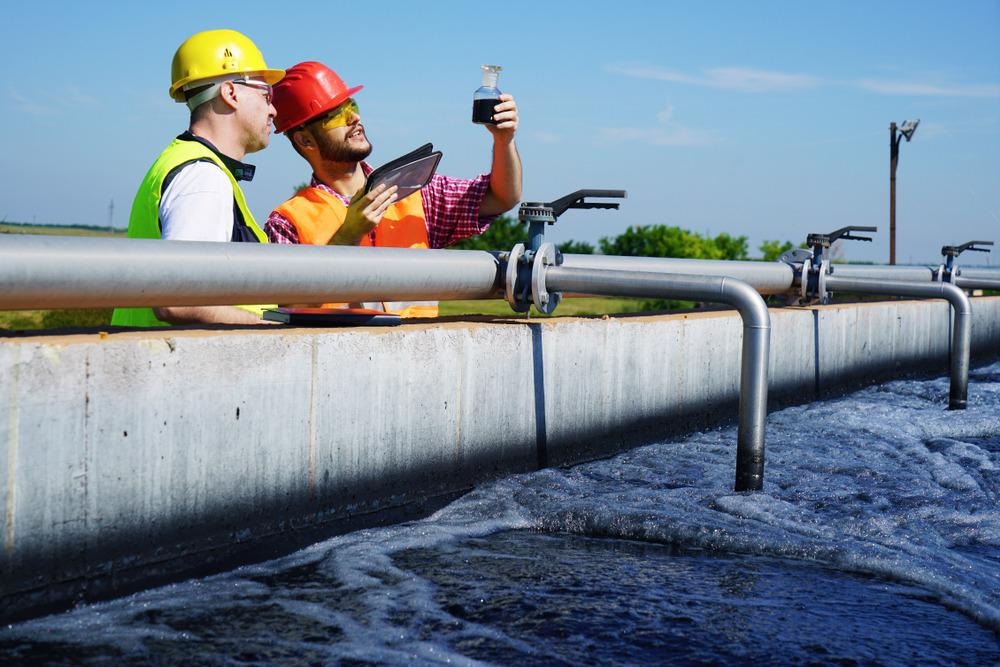
Image credit: Avatar_023/Shutterstock.com
Both municipal and industrial wastewaters present considerable health risks to human health and also endanger the environment; thus, all wastewaters must be carefully treated and closely monitored before being discharged.
As the public continues to push for human health and environmental protection, wastewater discharge regulations are becoming more strict. Each country possesses its own wastewater governing body and various discharge limits, resulting in the development and use of a diverse range of monitoring methods.
The requirement for rapid and accurate identification methods for contaminants is critical to the prevention of harmful discharge to public water sources.
The World Health Organization (WHO) came into being in 1948 to aid and promote global health.[6] In 2017, the WHO carried out a study involving 100 countries and 275 national standards relating to wastewater effluent quality requirements.
The study established the five most common groups of pollutants in wastewater as being chemicals, nutrients, organics, pathogens and solids, with organics being the category most frequently monitored.[28]
Organic compounds make up a considerable portion of wastewater contamination and have been monitored for over 100 years. The most frequently used analysis technique around the world to measure organic levels is Biochemical Oxygen Demand (BOD).[43]
As technology has progressed, regulations are permitting the use of other methods such as Chemical Oxygen Demand(COD)[44] and Total Organic Carbon(TOC)[45] for the evaluation of organic contamination.
Despite the extensive use of BOD, there is an emerging trend to move away from BOD/COD towards TOC in order to meet regulatory compliance as well as process control.
Parameters for Organic Contamination
Organic contamination is one category of pollution that needs to be monitored in wastewater due to its significance. However, because there is a multitude of organic compounds, it is not practical to measure each one of them singularly.
Thus, the concept of ‘sum parameters’ is applied to group together numerous compounds with similar qualities into a single category: BOD, COD and TOC are the most commonly used for organic contamination.
Biochemical Oxygen Demand
During the early part of the 20th Century, considerable levels of sewage and organic matter were being released into the River Thames, with an approximate five-day journey from Britain to the sea.
As microorganisms broke down the organic matter present, they also depleted dissolved oxygen levels in the water, harming aquatic life.[1, 48]
As a result, the five-day Biochemical Oxygen Demand (BOD5) test was invented in 1908 as a measure of organic pollution in water. BOD5 is one of the most frequently used sum parameters for establishing levels of organic contamination in wastewater.
The technique is dependent on microorganisms breaking down organic matter by consuming oxygen in the sample. Significant levels of organic matter in the water sample result in a greater expenditure of dissolved oxygen.
By measuring the consumption of oxygen consumed over a five-day incubation period at 20°C, the BOD5 test offers an indirect indication of organic contamination.[43]
The oxygen demand from the BOD test is generally a combination of carbonaceous biochemical oxygen demand (CBOD) and nitrogenous biochemical oxygen demand (NBOD), which takes place due to the decomposition of ammonia or other nitrogen-based compounds.
Nitrogenous demand can obstruct the BOD5 test, and so an alternative CBOD method is typically utilized, which requires the addition of an inhibitory compound.[43]
Due to the enduring acceptance of this test over the past century, BOD5 is a parameter that has been significantly incorporated into almost all global wastewater regulations. In spite of its pervasive use, there are a number of major issues with biochemical oxygen demand.
One major disadvantage of BOD5 is the five days needed between taking a sample and receiving results. This duration of this test makes BOD5 an impractical parameter for process control.[2, 8]
By the time a wastewater plant realizes that it has surpassed effluent discharge limits, it will have exceeded compliance control for several days.[42]
Another major disadvantage with the BOD5 test is the fact it relies on the growth of microorganisms. Thus, the presence of a compound which can impede biological growth, including chlorine, heavy metals, alkalinity or acidity, will affect the results.[8, 39]
BOD only measures what is naturally biodegradable, but there are several organic compounds that microorganisms cannot decompose, so BOD5 fails to identify all organic pollution in water.[8]
Due to the reliance on biological growth, the test not only encounters precision and accuracy issues[8, 42] but also demonstrates poor sensitivity.[42]
Chemical Oxygen Demand
Chemical Oxygen Demand (COD) is another indirect methodology that is used to establish the levels of organic contamination in wastewater. However, chemical oxidation is used during this test which breaks down the contamination in the water and then measures the oxygen that is exhausted in the process.
Similar to the BOD5 test, increased amounts of oxygen consumption usually stipulate higher levels of organics present in the sample.[3] There are numerous different methods of COD testing that have been approved.
The open reflux method necessitates that the sample is refluxed in strong acid in potassium dichromate. Volatiles may not be effectively oxidized due to short contact with oxidants.
Closed titrimetric reflux is a satisfactory method when the sample has increased levels of volatiles because they stay in contact with the oxidants for a prolonged period of time. Anything that can absorb visible light, such as insoluble suspended solids and color components, can impede the results.[44]
The COD test has some major advantages over BOD5. One significant benefit of the COD method is the reduced test duration. While BOD takes five days to acquire results, COD typically takes just a few hours.[2, 44]
Another benefit is that the test does not necessitate microbial growth for oxidation, therefore producing comparatively reliable and reproducible results.[2]
Unlike BOD, which can only determine the oxygen demand from biodegradable organics, COD oxidation is more complete and can oxidize almost all of the organics in the sample.
Thus, COD tests will have greater values but will also offer a more accurate evaluation of organic content in the water.
The major drawback with COD testing is the need to use toxic chemicals and subsequently produce more hazardous waste, including silver, hexavalent chromium and mercury: chlorides and other halides will interfere considerably with the test without the addition of silver or mercury ions.
Pyridine and similar aromatic compounds may repel oxidation and produce false low readings.[44]
Total Organic Carbon
Advances in technology over the years have brought about Total Organic Carbon (TOC) analyzers, which offer a direct method of measuring organic levels in water. Unlike BOD5 or COD, which use oxygen demand to establish organic levels, TOC analyzers measure and quantify the carbon present in the sample directly.[42, 44, 45]
All TOC analyzers function by oxidizing organic matter to CO2, which can then be measured using conductivity methods or non-dispersive infrared detection (NDIR).[45] Different methodologies of sample oxidation include combustion, UV persulfate and supercritical water oxidation (SCWO).[45]
TOC analysis has a number of advantages when compared to traditional oxygen demand tests. BOD5 can only measure oxygen demand from biodegradable organic material.
TOC analyzers oxidize all organic compounds rapidly to establish the organics present in the sample. Unlike COD testing, TOC analysis can identify the difference between organic carbon and inorganic carbon, including carbonate, bicarbonate and carbon dioxide.
If samples have reduced levels of volatile organics, analyzers can acidify and purge the inorganic carbon to quantify the non-purgeable organic carbon (NPOC).[43] Analyzers can also evaluate the Total Carbon (TC) and Total Inorganic Carbon (TIC) independently to calculate Total Organic Carbon.
Greater sensitivity and versatility are considerable advantages of TOC analyzers, which can identify organic concentration as low as 0.03 ppb and as high as 50,000 ppm.
In contrast to the conventional lab methods of BOD5 and COD, TOC yields accurate results in just a few minutes. TOC instruments are generally available in both lab and online models, which makes them indispensable tools for both regulatory compliance and process control.[43]
Standard Methods 5310 states, “Total Organic Carbon is a more convenient and direct expression of total organic content...measurement of TOC is of vital importance to operation of water treatment and waste treatment plants”.[45]
A Shift in Global Regulations for Organic Monitoring
Each region or country’s governing body establishes acceptable emission limits of organic contamination in wastewater discharge. BOD5, which has circulated since 1908, is included in almost all global regulations.
However, as the technology for monitoring advances, regulations are also evolving. Some countries permit the use of BOD to TOC correlations[4] or even state that TOC is used as a best available technology.[7]
Wastewater Regulations in North America
In 1999, the Canadian Environmental Protection Act (CEPA) was put in place to manage pollution and waste management.
Under the Fisheries Act, the Wastewater Systems Effluent Regulations were also passed.[13] Also known as SOR/2012-139, this document highlights effluent limits and details the conditions necessary for monitoring and reporting.
Current limits for organic contamination are detailed under the carbonaceous BOD parameter. [13, 34]
The SOR declares, “The demand due to the quantity of carbonaceous biochemical oxygen demanding matter in the effluent must be determined in accordance with a five-day biochemical oxygen demand test with nitrification inhibition.”[34]
The document identifies a CBOD limit of 25mg/L and declares that the operator must establish CBOD consistently on effluent samples, but that sample frequency can fluctuate depending on the plant size.[34]
In the United States, the Clean Water Act 1972 was developed due to the growing public concern for water pollution. This act authorized the US Environmental Protection Agency (USEPA) to determine wastewater standards and produce programs for pollution management.[17, 29]
The Clean Water Act led to the creation of the National Pollutant Discharge Elimination System (NPDES) to regulate point sources of pollutants being released. These permits have established requirements relative to discharge limits, monitoring and reporting.[26, 27]
Presently, BOD5 is classified as a conventional pollutant according to Section 304(a)(4) of the Clean Water Act.[22]
Although discharge requirements may differ based on industry and NPDES permitting, the Code of Federal Regulations 40 CFR 133.102 details the effluent limits for publicly owned treatment works (Table 1), stating, “At the option of the NPDES permitting authority, in lieu of the parameter BOD5...the parameter CBOD may be substituted”.[3]
Table 1. United States Discharge Limits for Publicly Owned Treatment Works. Source: SUEZ Water Technologies & Solutions
Parameter |
30-Day Average |
7-Day Average |
Removal |
5-Day BOD |
30 mg/L |
45 mg/L |
85% |
5-Day CBOD |
25 mg/L |
40 mg/L |
85% |
Despite the US NPDES permits identifying BOD5 a the standard test, 40 CFR 133.104 states, “Chemical oxygen demand (COD) or total organic carbon (TOC) may be substituted for BOD5 when a long-term BOD:COD or BOD:TOC correlation has been demonstrated”.[4]
Presently, numerous plants in the US have designed long term correspondence and now utilize TOC analysis to track their levels of wastewater discharge.[42]
Wastewater Regulations in Asia
The Ministry of Environmental Protection of the People’s Republic of China determines environmental policies and regulations for China.[25] The Integrated Wastewater Discharge Standard for China (GB8978-1996) was put in place to manage water pollution levels for health and the environment.
In 2002, the Ministry of Environment Protection released GB 18918-2002, which was developed especially for the control of wastewater treatment plant discharges.[49]
The regulations in China permit the use of both BOD and COD, with GB 8978-1996 identifying COD limits in industries such as pharmaceuticals and petrochemical. This regulation also identifies Total Organic Carbon limits for the synthetic fatty acid industry and the deluging industry.[20, 23]
Table 2 outlines the permissible wastewater discharge amount for pollutants across various industries.
Table 2. Allowable Wastewater Discharge Amount for Industries in China. Source: SUEZ Water Technologies & Solutions
Pollutant |
Application Scope |
Class 1 (mg/L) |
Class 2 (mg/L) |
BOD5 |
Beet Sugar, Dyes, Fur Treating, Can Sugar Processing, Alcohol |
20 |
60 |
Urban Secondary Wastewater Treatment Plants |
20 |
30 |
COD |
Pharmaceuticals |
100 |
300 |
Petrochemical/Refining |
60 |
120 |
Urban Secondary Wastewater Treatment Plants |
60 |
120 |
TOC |
Synthetic fatty acid industry |
20 |
40 |
Ramie degluing industry |
20 |
60 |
In September 1974, India’s Ministry of Environment, Forest, and Climate Change established the Central Pollution Control Board (CPCB) to manage pollution emissions in air and water.[5]
In 1986, The Bureau of Indian Standards (BIS) was created to incorporate a number of acceptable test methods and standards. In BIS 3025 Part 44, methods for Biochemical Oxygen Demand are detailed.
This standard state that in contrast to traditional BOD5 testing conducted at 20°C, three-day BOD testing conducted at 27° C is more suitable for hot climate conditions.[1] BIS 3025 Part 58 details appropriate methods for Chemical Oxygen Demand.
This standard highlights that the COD test is comparatively easy and accurate and has fewer interferences than BOD.[2]
Although India relies significantly on BOD measurements, the CPCB produced the “Guidelines for Online Continuous Effluent Monitoring Systems” (OCEMS), which debate TOC technology.
In section 4.6, this document declares, “TOC is a more convenient and direct expression of total organic content than either BOD or COD.” Similar to US guidelines, the document allows for TOC to be used to estimate accompanying BOD or COD “if a repeatable empirical relationship is established.” [16]
Wastewater Regulations in Europe
In 1991, the Urban Waste Water Treatment Directive (UWWTD) was created by the European Union (EU).
This document was developed to protect the environment from severe discharges from the urban wastewater treatment plants, food processing plants and rainwater run-off. Table 3 details the requirements of BOD and COD from urban wastewater treatment plants as stated in the document.
Table 3. Discharge Requirements from European Urban Wastewater Treatment Plants. Source: SUEZ Water Technologies & Solutions
Parameters |
Concentration |
Minimum percentage of reduction |
BOD5 at 20°C without nitrification |
25 mg/L O2 |
70-90 |
COD |
125 mg/L O2 |
75 |
The document stipulates, with regards to BOD5, “The parameter can be replaced by another parameter: total organic carbon (TOC) ... if a relationship can be established between BOD5 and the substitute parameter”.[14]
In 2000, The European Union issued the Water Framework Directive (2000/60/EC), which determined water quality goals and parameters for the European Union.[30]
In 2010 the Industrial Emissions Directive (2010/75/EU) was issued, which focused on the reduction of industrial emissions to the environment.This document establishes industry categories as energy, metal production, chemical and waste management. [15, 18]
In 2016, under Directive 2010/75/EU, document 2016/902 was disclosed to detail the best available methods (BATs) and relative emission limits (AELs) for wastewater in the industrial sector.
As per the Industrial Emissions Directive, these BAT-AEL practices were to be incorporated across a four-year period. This document determines TOC or COD should be monitored daily in compliance with EN standards.
It cites standard EN 1484 as the technique for measuring TOC. [7] Table 4 highlights the general BAT-AELs for direct emissions of TOC and COD to a receiving water body.
Table 4. European BAT-AELs for Direct Emissions of TOC and COD. Source: SUEZ Water Technologies & Solutions
Parameter |
BAT-AEL (yearly average) |
Conditions |
Total Organic Carbon (TOC) |
10-30 mg/L |
The BAT-AEL applies if the emission exceeds 3,3 t/yr |
Chemical Oxygen Demand (COD) |
30-100 mg/L |
This BAT-AEL applies if the emission exceeds 10 t/yr |
The document stipulates, “No BAT-AEL applies for Biochemical Oxygen Demand (BOD). As an indication, the yearly average BOD5 level in the effluent from a biological wastewater treatment plant will generally be ≤ 20 mg/l.”
It also mentions that either limit for TOC or COD applies but specifies “TOC is the preferred option because its monitoring does not rely on the use of very toxic compounds.”[7]
Developing TOC correlations to BOD5
Despite the BOD5 test being broad and non-specific, most regulatory bodies are apprehensive when it comes to replacing such a well-established industry standard.
However, some countries, including the US and India, know the value in other testing parameters and are permitting the application of BOD to TOC correlations.
As Standard Methods 5310A states, “If a repeatable empirical relationship is established between BOD, AOC, or COD for a specific source water then TOC can be used to estimate the accompanying BOD, AOC, or COD. This relationship must be established independently for each set of matrix conditions”.[42, 45]
Producing BOD to TOC correlation typically requires collaboration with the local governing body to design a long-term study.
As BOD5 results tend to be vague at best and several data points are needed to produce information appropriate for the development of this correlation and the subsequent regression curve equation.
The permitting or governing body must sign off on the correlation. A number of plants across the US have developed site-specific correlations and now make use of TOC to monitor their wastewater discharges. [16, 42]
Inland Empire Utilities Agency, a San Bernardino County-based wastewater treatment facility, uses TOC to monitor its water.
The NPDES Issuance and Waste Discharge permit issued to Inland Empire stipulates, “the Discharger has demonstrated a correlation between the biological oxygen demand (BOD5) and total organic carbon (TOC) concentrations in the effluent to the satisfaction of the Executive Officer.”[12]
This enables Inland Empire to establish BOD5 compliance predicated on TOC analysis. For influent monitoring and daily for tertiary effluent monitoring, the permit necessitates a weekly composite of BOD and TOC, stating “BOD5 is calculated based on a BOD /TOC correlation approved by the Regional Water Board”.[12, 31]
The City of Santa Cruz in California has also established a long-term TOC correlation study for its wastewater treatment plant. The NPDES
Water Discharge Requirements document, which highlights the plant’s effluent limitations for traditional pollutants, declares “the discharger has demonstrated an adequately robust statistical correlation between TOC and BOD at this facility”[32] and is approved to utilize a TOC correlation to meet the BOD5 effluent limitations.
The approved site-specific TOC correlation for the City of Santa Cruz was established to be:
TOC = 0.4141(BOD ) + 4.3937. Table 5 exhibits the approved TOC limits based on the correlation. [32, 36]
Table 5. City of Santa Cruz - Average Weekly and Monthly Discharge. Source: SUEZ Water Technologies & Solutions
Parameter |
Units |
Average Monthly |
Average Weekly |
TOC |
mg/l |
17 |
23 |
The article titled “Faster and Smarter” posted by the city of Santa Cruz states, “This study demonstrates the feasibility of developing site-specific TOC values for effluents by publicly owned treatment works.
The development also enhanced efficiency in operations by enabling the substitution of TOC analyses for BOD in plant process control because of the shorter turnaround times”.[36]
New Trends in TOC Analysis for Process Control
Process Control & Asset Protection
It is critical for regulatory compliance to measure organic loading at final discharge. However, monitoring organics at the flow point and during treatment has emerged as an effective practice for process control and asset optimization.
For instance, direct influent monitoring of carbon at municipal wastewater treatment plants can help enhance the biological treatment, leading to optimized process control and the ability to make process decisions in real-time.
TOC analysis is gaining approval as a tool to improving the durability of water treatment assets.
As filtration membranes are increasingly utilized by industrial and water reuse plants to treat wastewater, TOC analyzers can be deployed to rapidly detect high organic loading, therefore, limiting fouling and facilitating treatment efficiency evaluation.
In addition, a number of plants are merging biological treatment and membrane filtration in a process referred to as membrane bioreactors (MBR). Direct carbon monitoring in the MBR influent enables plants to optimize the biological treatment and shields membranes against organic fouling.
Optimal Food to Microorganism Ratio
Municipal plants treat influent wastewater following a number of steps. The primary treatment entails physical separation, whereby solids are extracted by screening and sedimentation.
Subsequent to this primary treatment, plants generally use a secondary biological treatment process to limit the organic content of influent wastewater.[47]
This process usually depends on the use of aerobic bacteria in activated sludge to help disintegrate organic compounds found in the water. Organic molecules, which are frequently measured by the traditional BOD test, serve as ‘food’ for the bacteria.[35]
To ensure a suitable balance of organics and microorganisms in the treatment, plants use a common parameter known as food to microorganism (F:M) ratio.[21]
A system with a low F:M ratio signifies insufficient ‘food’ and leading to starvation of the microorganisms responsible for the breakdown of organic molecules. However, microbes will likely be overburdened by the organic loading in a system with a high F:M ratio. This can lead to ineffectual removal of organic contaminants.
To maximize the health of the biomass and guarantee removal of organic contamination, it is key that plants operate at their optimal F:M ratio.
Unlike conventional oxygen demand tests, TOC analyzers measure the amount of carbon present in the wastewater directly, allowing operators to quantify the ‘food’ in their F:M ratio with good accuracy.
The five-day response time for the BOD5 test is typically not fast enough for process adjustments, especially in plants with fluctuating organic loading.
To accelerate response time to the fluctuating organic loads of influent wastewater, a number of plants are moving towards TOC analysis, which offers rapid analysis without the need for hazardous chemicals.
The capacity to make high speed process adjustments with TOC analysis while measuring the carbon fed to the system directly enables plants to sustain an optimal F:M ratio, making sure the biological treatment performs at an ideal functionality.
UF & RO Membrane Optimization
The ability to detect organic carbon directly and rapidly has also enabled TOC analysis to become a reliable tool for membrane protection in wastewater treatment plants, especially in regions with limited water sources.
These water-scarce regions have initialized the use of ultrafiltration (UF) and reverse osmosis (RO) membranes to treat wastewater for reuse.[41] [46]
In membrane filtration, contaminated water is fed through a semipermeable membrane, which divides suspended solids and large molecular weight compounds from the industrial effluent.
However, significant levels of organic contamination in water streams often lead to organic fouling by amassing on membrane surfaces, and some compounds can result in membrane damage.
Increased fouling of membranes leads to a reduction in the flux of liquid across the membrane and reduces the effectiveness of the treatment.
While increasing transmembrane pressure (TMP) to sustain the appropriate flux across fouling membranes can be effective[41], it tends to lead to an increase in energy costs. Repairing or replacing fouled membranes can restrict the operating capacity of the wastewater treatment plant and thereby also inflate costs.
Although backflush and chemical clean-in-place (CIP) strategies are applied on a routine basis, frequent cleaning cycles are often required for membranes that process waters with high carbon loading.[41]
Not only does this cause increased downtime and higher incur higher costs for cleaning chemicals, but it reduces the lifespan of membranes.
To guarantee longevity of membranes and function at prime efficiency, it is advantageous for plants to track organic content levels of the water directly upstream of the membranes.
While traditional oxygen demand tests can offer an indirect indication of contamination levels, TOC analysis supplies immediate data on the carbon loading of the wastewater without impedance.
This allows plants to adjust flows to both protect membranes but also evaluate treatment effectiveness and determine process upsets upstream. Online TOC analysis of water before and after membranes offers real-time data of carbon loading and extraction efficiency across the membranes over time.
By moving away from oxygen demand and towards TOC analysis, a number of plants are finding an improvement in economic savings through asset protection.
MBR Optimization
Membrane Bioreactor (MBR) systems are a treatment process that that is attracting attention in both municipal and industrial wastewater plants. This process merges both biological treatment and filtration membranes to limit the number of organics in wastewater.
The advantages MBR systems provide a considerably smaller footprint than traditional biological treatment, increased pathogen removal and higher grade effluent.
Akin to traditional biological treatment, the wastewater in MBR systems is initially introduced to an aerated tank with activated sludge. Before being introduced to a membrane that is submerged in the water, the microbes in the sludge begin to break down the organic contamination in the sample (microfiltration or ultrafiltration). [37]
The water is fed through the membrane, which not only extracts additional contamination but also rejects any solids that arise during the biological process. This blend of biological treatment and submerged filtration membranes usually results in cleaner effluent water than what would result from a singular process.
As with other membrane filtration systems, fouling can be a considerable factor for MBR systems.[41] They may endure clogging and sludging, which requires increased downtime and maintenance.
MBR systems, like traditional biological treatment, depend on sustaining an optimum F:M ratio to guarantee efficient organic removal. Optimizing the F:M ratio is a productive method that helps mitigate any MBR membrane-associated risk.
By running at an optimum F:M ratio on a consistent basis, plants can guarantee the health of the biomass [37] as well as limiting organics that may contribute to membrane fouling.
Although the organic levels for F:M ratio is traditionally measured as BOD5, plants are now converting to online TOC analyzers for high speed, direct measurements of the carbon in the water.[11]
Through facilitating immediate process decisions, operators can sustain an optimal F:M ratio, which reduces costs and maintenance of fouled membranes.
The capacity of TOC analysis to measure carbon loading directly at high speeds is propelling organics analysis past regulatory discharge compliance and into cost reduction via process control and asset protection.[35]
Conclusion
Currently, BOD5 is the most popular parameter in use for organic pollution in industrial wastewaters. Despite its lack of precision and many impedances, it has been incorporated into global wastewater regulations.
While COD testing is much faster and more precise, it requires the use and disposal of extremely toxic chemicals.
TOC analyzers are increasingly gaining popularity with the capacity to produce rapid and accurate data in just a matter of minutes. Unlike BOD5 and COD tests, TOC analyzers measure organic levels directly rather than indirectly establishing the organic content by measuring oxygen demand.
Numerous regulatory bodies now see the value of state-of-the-art technologies such as TOC.
Presently, the United States has authorized plants to use TOC instead of BOD if a prolonged correlation study has been carried out and approved. An example of the transformation in test method is the European Union, which no longer recommends BOD5, but instead focuses on TOC because of the lack of toxic chemicals.
As Europe moves from antiquated test methods, other countries are starting to realize the benefits of a transformation in monitoring processes and a change in regulations.
Governing bodies around the world will continue to introduce more accurate and precise parameters into the regulations along with advances in technology. In a world where industrial growth continues to expand, the necessity to monitor wastewater accurately has never been more critical.
The power of TOC for regulatory monitoring, asset protection and process control allow plants to move toward a future of exemplary monitoring.
References
- “3025 Part 44 - Biochemical Oxygen Demand.” Bureau of Indian Standards, https://archive.org/details/gov.law.is.3025.44.1993/page/n3
- “3025 Part 58 - Chemical Oxygen Demand.” Bureau of Indian Standards, https://archive.org/details/gov.law.is.3025.58.2006/page/n3
- “40 CFR 133.102 Secondary Treatment.” Electronic Code of Federal Regulations, https://www.law.cornell.edu/cfr/text/40/133.102
- “40 CFR 133.104.” Electronic Code of Federal Regulations, https://www.ecfr.gov/cgi-bin/text-idx?SID=4f99ad02644fb790819e9af0dabed218&mc=true&node=pt40.24.133&rgn=div5#se40.24.133_1104
- “About Us.” Central Pollution Control Board, https://cpcb.nic.in/
- “About WHO.” World Health Organization, https://www.who.int/about/who-we-are
- “BAT 2016/902” https://eur-lex.europa.eu/legal-content/EN/TXT/PDF/?uri=CELEX:32016D0902&from=EN
- “Biochemical Oxygen Demand (BOD).” Pennsylvania Department of Environmental Protection, https://www.watereducation.org/
- “Biochemical Oxygen Demand and Chemical Oxygen Demand.” Caltest Analytical Labs, https://www.caltestlabs.com/Services/BODandCOD.aspx
- “Biological Oxygen Demand.” Encyclopedia of Public Health, Encyclopedia.com, 2019, https://www.encyclopedia.com/science-and-technology/biology-and-genetics/environmental-studies/biological-oxygen-demand#biologicaloxygendemand.
- “Bottling company uses Sievers InnovOx Online TOC analyzer to Optimize Membrane Bioreactor Wastewater System” Suez Water Technologies and Solutions. https://www.suez.com/en
- “California Regional Water Quality Control Board NPDES Permit Order No Ca8000409.” Inland Empire Utility Agency, 20 July 2009, https://www.ieua.org/
- “Canadian Environmental Protection Act Registry.” Government of Canada, 24 April 2019, https://www.canada.ca/en/environment-climate-change/services/canadian-environmental-protection-act-registry.html
- “Council Directive concerning urban wastewater treatment (97/271/EC).” Official Journal of the European Communities, https://eur-lex.europa.eu/legal-content/EN/TXT/PDF/?uri=CELEX:31991L0271&from=EN
- “Directive 2010/75/EU Of European Parliament on Industrial Emissions.” 24 November 2010, https://eur-lex.europa.eu/legal-content/EN/TXT/?uri=celex%3A32010L0075
- “Guidelines for Online Continuous Effluent Monitoring.” Central Pollution Control Board, July 2018
- “History of the Clean Water Act.” United States Environmental Protection Agency, 8 August 2017, https://www.epa.gov/laws-regulations/history-clean-water-act
- “Industrial Emissions Directive.” European Commission, 25 March 2019, http://ec.europa.eu/environment/industry/stationary/ied/legislation.htm
- “Industry Effluent Standards.” Central Pollution Control Board, http://cpcb.nic.in/industry-effluent-standards/
- “Integrated Wastewater Discharge Standard – GB 8978-1996.” National Standard of the people’s republic of China, Chinese Standard, https://www.chinesestandard.net/PDF.aspx/GB8978-1996
- “Introduction to Activated Sludge.” Wisconsin Department of Natural Resources. December 2010 https://dnr.wi.gov/regulations/opcert/documents/WWSGActSludgeINTRO.pdf
- “Learn about Effluent Guidelines.” United States Environmental Protection Agency, 21 November 2018, https://www.epa.gov/eg/learn-about-effluent-guidelines
- “Maximum Allowable Discharge Concentrations for Other Pollutants in China.” China Water Risk, https://www.chinawaterrisk.org/
- “Method 410.3: Chemical Oxygen Demand (Titrimetric, High Level for Saline Waters) by Titration.” United States Environmental Protection Agency, https://www.epa.gov/sites/production/files/2015-08/documents/method_410-3_1978.pdf
- “Ministry of Ecology and Environment of the People’s Republic of China.” www.mee.gov.cn
- “National Pollutant Discharge Elimination System (NPDES) – About NPDES." United States Environmental Protection Agency, 29 November 2016, https://www.epa.gov/npdes/about-npdes
- “National Pollutant Discharge Elimination System (NPDES) – NPDES Permit Basics." United States Environmental Protection Agency, 25 July 2018, https://www.epa.gov/npdes/npdes-permit-basics
- “Progress on Wastewater Treatment.” World Health Organization, 2018, https://www.who.int/
- “Summary of the Clean Water Act 33 U.S.C. §1251 et seq. (1972).” Laws and Regulation, United States Environmental Protection Agency, 11 March 2019, https://www.epa.gov/laws-regulations/summary-clean-water-act
- “The EU Water Framework Directive.” European Commission, 6 August 2018, https://environment.ec.europa.eu/topics/water/water-framework-directive_en
- “Title 22 Engineering Report.” Inland Empire Utilities Agency, January 2010, https://www.ieua.org/
- “Waste Discharge Requirements for the City of Santa Cruz Wastewater Treatment Plant.” California Regional Water Quality, https://www.waterboards.ca.gov/rwqcb3/board_decisions/adopted_orders/2010/2010_0043_Santa_Cruz.pdf
- “Wastewater Regulations Overview.” Government of Canada, 7 February 2007, https://www.canada.ca/en/environment-climate-change/services/wastewater/regulations.html
- “Wastewater Systems Effluent Regulations SOR/2012-139”. Justice Laws Website, Government of Canada, https://laws-lois.justice.gc.ca/eng/regulations/sor-2012-139/fulltext.html
- Assman, Celine, et al. “Online total organic carbon (TOC) monitoring for water and wastewater treatment plants processes and operations optimization.” Drinking Water Engineering and Science. August 2017 https://www.researchgate.net/publication/318976763_Online_total_organic_carbon_TOC_monitoring_for_water_and_wastewater_treatment_plants_processes_and_operations_optimization
- Baba, Akin and Tianfei Xu. “Faster and Smarter - A BOD-to-TOC conversion enables quick response to process control needs.” City of Santa Cruz Water environment laboratory October/November 2010, http://www.cityofsantacruz.com/home/showdocument?id=21451
- Bengtson, Harlan H. “Biological Wastewater Treatment Processes III: MBR Processes.” CED Engineering https://www.cedengineering.com/userfiles/02%20-%20Biological%20WWTP%20III%20-%20Membrane%20Bioreactor.pdf
- Bengtson, Harlan H, “Biological Wastewater Treatment Processes III: MBR Processes” CED Engineering.com https://www.cedengineering.com/userfiles/02%20-%20Biological%20WWTP%20I%20-%20Activated%20Sludge.pdf
- Delzer, G.C. and S.W. McKenzie. “Five Day Biochemical Oxygen Demand.” United States Geological Survey, November 2003, https://water.usgs.gov/owq/FieldManual/Chapter7/NFMChap7_2_BOD.pdf
- Hazma, Rania Ahmed, et al. « Membrane Bioreactor (MBR) Technology for Wastewater Treatment and Reclamation: Membrane Fouling.” Membranes (Basel). June 2016. https://www.ncbi.nlm.nih.gov/pmc/articles/PMC4931528/
- Muro, Claudia, et al. “Membrane Separation Process in Wastewater Treatment of Food Industry.” Institute Technology of To luca
- Nutt, Stephen G. and John Tran of XCG Consultants Ltd. “Addressing BOD5 limitations through Total Organic Carbon Correlations: A Five Facility International Investigation.” Pensacola, Florida: water & Wastewater Instrumentation Testing Association of North America (ITA). January 2013.
- Rice, E.W. et al. “5210 Biochemical Oxygen Demand.” Standard Methods for the Examination of Water and Wastewater. 22nd ed. 2012. Washington, DC: American Water Works Association. Print.
- Rice, E.W. et al. “5220 – Chemical Oxygen Demand.” Standard Methods for the Examination of Water and Wastewater. 22nd ed. 2012. Washington, DC: American Water Works Association, Print.
- Rice, E.W. et al. “5310 – Total Organic Carbon” Standard Methods for the Examination of Water and Wastewater. 22nd ed. 2012. Washington, DC: American Water Works Association, Print.
- Shon, H.K et al. “Membrane technology for organic removal in wastewater.” Faculty of Engineering, University of Technology, Sydney Australia, Dec 2007 https://pdfs.semanticscholar.org/0818/e843ada017587afdc653a438fe45801b6614.pdf (D)
- Toit, Wynand du. “Use of total organic carbon on a wastewater treatment plant.” Tshwane University of Technology, September 2006 http://citeseerx.ist.psu.edu/viewdoc/download?doi=10.1.1.608.8456&rep=rep1&type=pdf
- Zainudin, Zaki bin. “The Many Intricacies of Biochemical Oxygen Demand.” Research Gate, January 2008, https://www.researchgate.net/publication/271019944_The_Many_Intricacies_of_Biochemical_Oxygen_Demand
- Zhou, Yuhua, et al. “COD Discharge Limits for Urban Wastewater Treatment Plants in China Based on Statistical Methods” Agricultural Green Infrastructure for Nutrient Reduction in Watersheds – Volume 10, Issue 6, June 2018. https://www.mdpi.com/2073-4441/10/6/777/htm
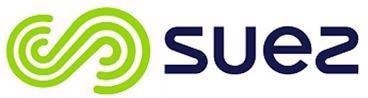
This information has been sourced, reviewed and adapted from materials provided by SUEZ Water Technologies & Solutions.
For more information on this source, please visit SUEZ Water Technologies & Solutions.