Drones have come a long way in the last decade, showing up everywhere from farms to disaster zones. But no matter how advanced they get, one thing continues to hold them back: battery life. Most UAVs (unmanned aerial vehicles) can only stay in the air for so long before they need to recharge, which limits what they can do and where they can go.
That’s where energy harvesting comes in. By pulling power from the environment, drones can extend their missions, run more sensors, and operate in places where swapping batteries just isn’t practical. Let’s take a closer look at how this tech is helping drones fly longer and smarter.
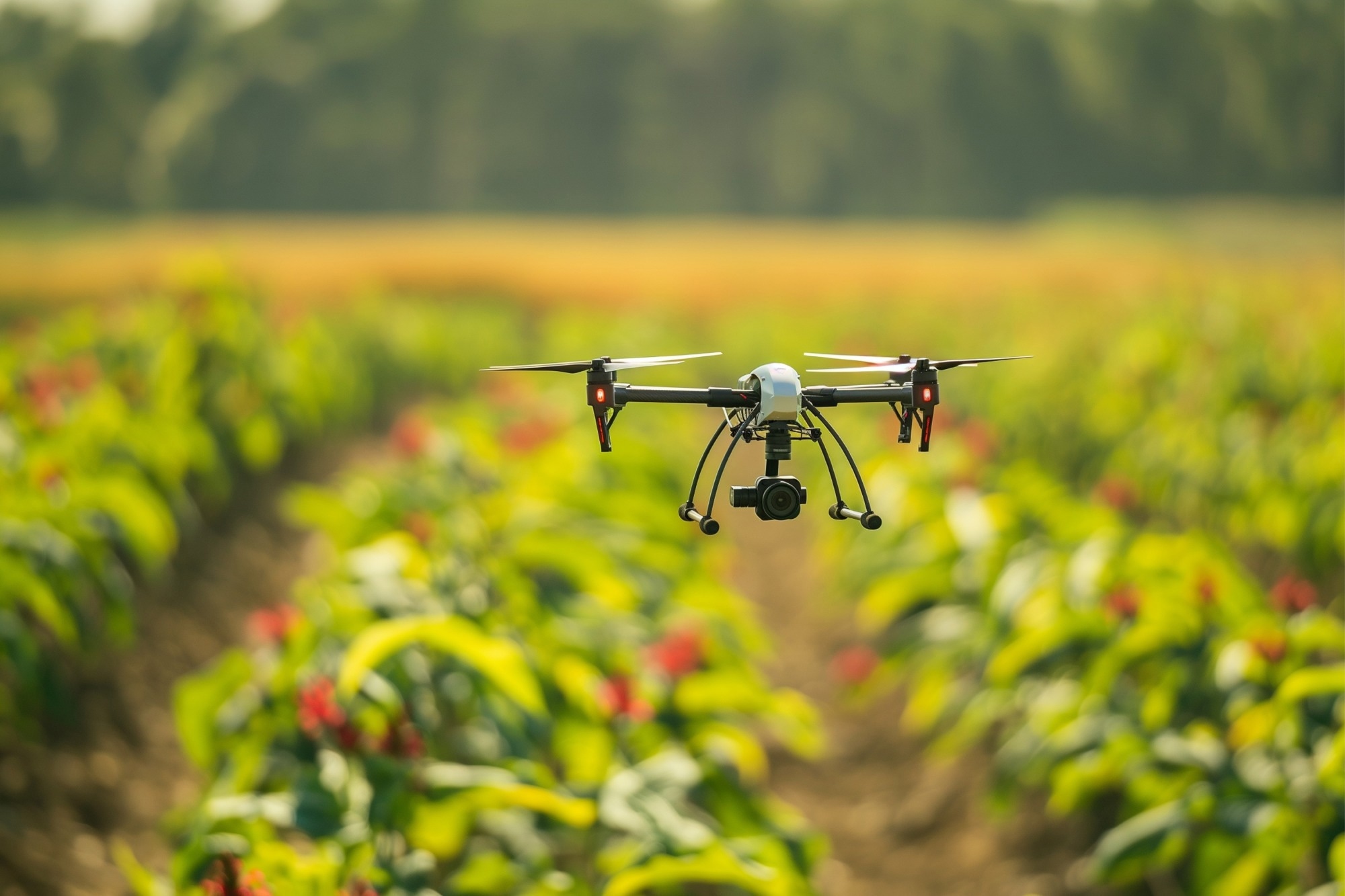
Image Credit: NovitaSari Image/Shutterstock.com
Download your PDF copy now!
Why Autonomy Demands Better Power Solutions
Autonomous drones need a continuous energy supply—not just for propulsion and flight stabilization, but also for powering onboard sensors, navigation systems, and data processing. While current battery tech offers decent capacity, the trade-off between weight, volume, and runtime often constrains mission duration and payload options.
Energy harvesting offers a complementary pathway. By tapping into environmental sources, drones can extend their operating window and reduce the need for manual recharging or battery swaps.1,2
One standout example is a battery-free, solar-powered fixed-wing UAV described in Scientific Reports. It uses lightweight photovoltaic cells for daytime flight and stores excess energy in supercapacitors to stay operational after sunset. This kind of design drastically reduces the need for maintenance, and when paired with low-power sensor systems, it opens the door to fully autonomous, long-range missions.2,3
Mechanical harvesting technologies are also catching up. Some experimental drones embed piezoelectric materials into their wings or fuselage, converting wing flapping or environmental vibrations into energy for small, real-time sensors, allowing continuous data collection with minimal onboard storage or power draw.³
Solar Energy Harvesting: Still the Most Practical Option
Solar remains the most mature and widely adopted form of energy harvesting in UAVs—and for good reason. Lightweight, flexible thin-film photovoltaics are now efficient enough to be integrated directly into drone surfaces without drastically impacting aerodynamics or payload capacity.
Take high-altitude pseudo-satellites (HAPS) like the Zephyr. These platforms operate for weeks at a time using only solar energy, serving roles in atmospheric data collection, communications relays, and persistent surveillance.4
On the lower end of the altitude spectrum, solar-powered drones are proving their worth in precision agriculture. One recent project combined supercapacitor-based energy storage with a lightweight, solar-only powertrain to enable takeoff, cruise, and landing—no battery required. Smart control algorithms onboard help manage variable irradiance, allowing the drone to dynamically adjust flight patterns based on available sunlight.2,4
This level of adaptability is particularly useful for large-scale agricultural monitoring, where sensor data needs to be collected over wide, often rugged, terrain, without relying on infrastructure or frequent human intervention.
Mechanical Harvesting: Extracting Power from Motion
Mechanical energy harvesting taps into the UAV’s own movement or environmental dynamics. There are two main approaches:
-
Wind-Induced Vibration: Piezoelectric harvesters embedded in wing structures or compliant surfaces can convert low-frequency aerodynamic vibrations into power. In optimal conditions, systems have been shown to generate up to 640 µW, enough to run localized pressure or temperature sensors.1,3
-
Flapping-Wing UAVs: Inspired by natural flyers, some biomimetic drones use wing oscillations not just for thrust, but also for generating electricity. One prototype uses a hybrid triboelectric-electromagnetic harvester to simultaneously measure and optimize performance while generating enough energy to power lightweight electronics.1
These systems shine in micro aerial vehicles (MAVs), where battery weight can make up over half the total mass. By replacing or supplementing batteries with harvesters, MAVs become more viable for tasks like tunnel inspection, indoor navigation, or missions where recharging isn’t an option.3
Wireless Energy: RF and Inductive Harvesting
Harvesting ambient RF signals or using inductive coupling provides UAVs with an alternative power strategy, especially when interacting with ground-based or aerial sensor networks.
One agricultural use case involves UAVs transmitting RF signals to power embedded soil moisture sensors. These sensors harvest energy from the drone’s RF field, collect data, and return it via BLE—no buried wires, no battery replacements.5
Another example: drones equipped to install and use inductive charging stations mounted on power lines. A team demonstrated a system that harvests up to 145 W from the magnetic field of overhead lines, allowing drones to recharge in place during extended grid inspections.6 These systems reduce both cost and risk by minimizing the need for human crews in hazardous conditions.
Smarter Sensor Systems Through Energy Harvesting
Energy harvesting doesn’t just extend flight time—it enables more ambitious sensing applications. Here’s how it’s changing the game:
- Endurance + Sensors: Solar-powered UAVs with multispectral or thermal imaging sensors can conduct continuous monitoring of crop health, forest fires, or wildlife patterns.4
- Noise Reduction: Eliminating wired power reduces electrical noise. For example, self-powered vibration sensors offer cleaner data when inspecting turbines or bridges.1
- Networked Data Collection: RF-powered sensor tags let drones scan large areas, collecting localized data from hundreds or even thousands of nodes in a single flight.7
This kind of scalable, low-maintenance sensing architecture is a big step toward real-time, data-rich decision-making in sectors like precision agriculture, smart cities, and environmental science.
Where It's Already Working
Energy harvesting-equipped drones are solving real-world challenges across sectors.
- Precision Agriculture: Farmers are using solar-powered drones equipped with RF harvesters to monitor soil health efficiently. These drones operate during daylight, utilizing solar energy to power multispectral cameras while wirelessly charging underground RF-enabled soil sensors. This setup allows for real-time data transmission regarding moisture and nutrient levels, enabling precise irrigation recommendations.7
- Disaster Response: In the aftermath of natural disasters, drones fitted with piezoelectric vibration harvesters navigate windy areas to assess flood damage. The harvesters convert turbulent airflow into energy, allowing thermal cameras to locate survivors trapped under debris. These drones can operate for extended periods without needing battery replacements, significantly outperforming traditional UAVs.8
- Wildlife Conservation: Researchers employ bio-inspired flapping-wing drones to monitor poaching activities in natural habitats. The wing motion generates energy through electromagnetic harvesters, which power acoustic sensors designed to detect gunshots. This self-sustaining system operates quietly, ensuring minimal disturbance to wildlife while providing timely alerts to rangers.9
- Smart Cities: Drones are also being employed for infrastructure inspection by harnessing energy from electromagnetic fields generated by streetlights. They are designed to dock on lampposts with inductive charging pads during nighttime patrols, recharging their batteries while conducting traffic light and bridge inspections, substantially reducing costs and enabling continuous operations.7
- Marine Biology: Autonomous drones equipped with hydrodynamic turbines can transform ocean monitoring. As they skim across the water's surface, the turbines can capture wave energy, powering sensors that measure salinity and temperature, providing crucial data on environmental changes.10
- Healthcare Delivery: Hybrid drones can be used to deliver medicines and vaccines. During flight, piezoelectric strips could harvest energy from rotor vibrations, keeping refrigeration units operational to maintain vaccine stability in remote areas.1,11
Challenges and Limitations
Energy harvesting technologies, while promising, encounter several significant challenges and limitations. One major hurdle is their dependence on weather conditions; for instance, solar-powered drones struggle to operate effectively in cloudy or low-light environments, which can severely limit their functionality during certain times and in different climates. Similarly, wind-based energy systems can fail to produce adequate power in calm weather, further complicating their reliability.2,4
Another issue is the trade-off between weight and energy harvesting capabilities. Integrating devices such as piezoelectric patches or solar panels can increase energy capture, but often compromise the payload capacity of drones, which in turn restricts the range of sensors and equipment that can be utilized for various applications.3
Furthermore, current triboelectric nanogenerators exhibit efficiency rates of less than 10%, highlighting the need for more effective solutions. To tackle these challenges, researchers are developing adaptive algorithms like Maximum Power Point Tracking (MPPT), which enhance energy capture by optimizing performance under fluctuating conditions.1,6
Looking Ahead: Smarter Systems, Smarter Drones
The future of UAV energy management lies at the intersection of energy harvesting and intelligent systems. By integrating artificial intelligence (AI) and machine learning (ML), drones can forecast energy availability and make real-time decisions, like adjusting flight paths to maximize exposure to sunlight during overcast conditions.4 These adaptive strategies help drones optimize power usage and improve mission reliability in unpredictable environments.
At the same time, advances in materials science are pushing the boundaries of what's possible. Emerging technologies like plasmonic nano-antennas could enable drones to harvest energy from infrared radiation, extending their operational capacity beyond the limits of traditional solar harvesting. And rather than relying on a single energy source, hybrid systems are gaining traction.
By combining solar, mechanical, and RF harvesting methods, UAVs can maintain power continuity across varying conditions. One promising example is the flapping-wing drone equipped with both solar cells and piezoelectric strips, offering redundancy and resilience in flight.1,3
Energy harvesting isn’t just a workaround for battery limitations; it’s becoming a cornerstone of next-generation UAV design. As research continues and adoption grows, we’re moving closer to a future where drones are self-sustaining: operating longer, collecting richer data, and requiring less manual support. The shift toward smarter, greener aerial systems is well underway, and energy harvesting will be a key part of making that future real.
Want to Learn More?
If you're interested in the future of autonomous drones and sustainable aerial tech, here are a few topics worth exploring next:
Download your PDF copy now!
References and Further Reading
- Wei, X. et al. (2023). Energy harvesting fueling the revival of self-powered unmanned aerial vehicles. Energy Conversion and Management, 283, 116863. DOI:10.1016/j.enconman.2023.116863. https://www.sciencedirect.com/science/article/pii/S0196890423002091
- Liller, J. et al. (2025). Development of a battery free, solar powered, and energy aware fixed wing unmanned aerial vehicle. Scientific Reports, 15(1), 1-18. DOI:10.1038/s41598-025-90729-2. https://www.nature.com/articles/s41598-025-90729-2
- Citroni, R., Di Paolo, F., & Livreri, P. (2019). A Novel Energy Harvester for Powering Small UAVs: Performance Analysis, Model Validation and Flight Results. Sensors, 19(8), 1771. DOI:10.3390/s19081771. https://www.mdpi.com/1424-8220/19/8/1771
- Noor, U. (2024). Solar-Powered Drones (2025). 8MSolar. https://8msolar.com/solar-powered-drones/
- Singh, A. (2024). The Role of Energy Harvesting in Advancing Sensor Systems. AZoSensors. https://www.azosensors.com/article.aspx?ArticleID=3114
- Muñoz-Gómez, A. M. et al. (2022). A Novel Charging Station on Overhead Power Lines for Autonomous Unmanned Drones. Applied Sciences, 13(18), 10175. DOI:10.3390/app131810175. https://www.mdpi.com/2076-3417/13/18/10175
- Veloo, S. G. et al. (2022). A Hybrid Solar-RF Energy Harvesting System Based on an EM4325-Embedded RFID Tag. Electronics, 12(19), 4045. DOI:10.3390/electronics12194045. https://www.mdpi.com/2079-9292/12/19/4045
- Pokhrel, S. R. (2020). Federated learning meets blockchain at 6G edge: a drone-assisted networking for disaster response. In MobiCom '20: The 26th Annual International Conference on Mobile Computing and Networking. ACM. DOI:10.1145/3414045.3415949. https://dl.acm.org/doi/abs/10.1145/3414045.3415949
- Team, N. (2025). Mara Elephant Project Drone Technology. Explore the Best of East Africa | Nomad Africa Travel Agency. https://nomad.africa/inspiration/mara-elephant-project-drone-technology
- Acharya, B. S. et al. (2021). Unmanned Aerial Vehicles in Hydrology and Water Management: Applications, Challenges, and Perspectives. Water Resources Research, 57(11), e2021WR029925. DOI:10.1029/2021WR029925. https://agupubs.onlinelibrary.wiley.com/doi/full/10.1029/2021WR029925
- Aggarwal, S. et al. (2024). Assessing the Feasibility of Drone-Mediated Vaccine Delivery: An Exploratory Study. Health Science Reports, 8(1), e70208. DOI:10.1002/hsr2.70208. https://onlinelibrary.wiley.com/doi/10.1002/hsr2.70208
Disclaimer: The views expressed here are those of the author expressed in their private capacity and do not necessarily represent the views of AZoM.com Limited T/A AZoNetwork the owner and operator of this website. This disclaimer forms part of the Terms and conditions of use of this website.