By Kalwinder KaurJan 2 2013
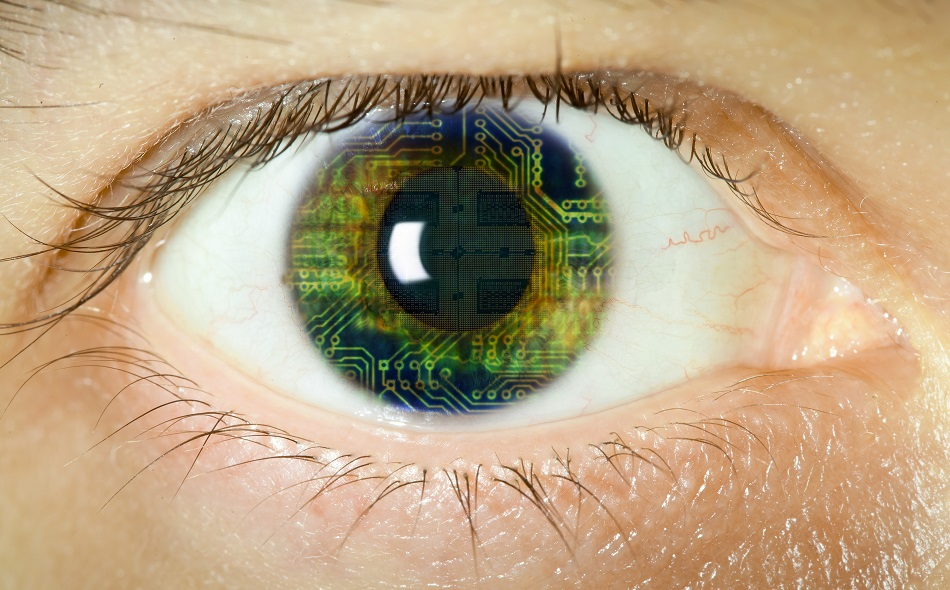
Image Credit: nexus 7/Shutterstock.com
Since the 1930s, ophthalmologists like Carl Foerster have been dedicated to developing advanced vision prostheses. As sensor technology continues to improve, research scientists have incorporated this technology into the recent expansion of optical sensory prostheses.
Human Sight
The human eye is critical in identifying the electromagnetic radiation from the visible spectrum and converting it into chemically mediated nervous impulses that will be perceived by the nervous system. However, this conversion occurs in the outer surface of the rods and cones of the human eye. The rods and cones are vital cells that can collect photons from the visible spectrum using visual pigments present within them. These pigments are regenerated inside the cells by a cyclic metabolic pathway that in turn provides a continuous response.
The first response tends to change the charge on the surface of a light-sensitive cell, which is converted into nerve impulses. Color vision is related to a series of chemical changes occurring in cone cells.
The following video by the University of New South Wales is presented by Professor Nigel Lovell and discusses the development of a working bionic eye, a concept that may soon be a reality.
Design and Development
The rods and cones of individuals suffering from macular degeneration, retinitis pigmentosa or other retinal disease are defective. Here, artificial retina prostheses are used for vision restoration. Schwiebert L. et al (2002) developed a novel system-smart sensor for restoring the vision of visually impaired people.
A smart sensor consists of a micro-bump array that is provided with extrusions through which the sensor rests on a retinal surface. The extrusions in each micro-bump array are provided with a small spacing to dissipate heat. The sensor is attached to an integrated circuit equipped with pads and on-chip switches for supporting connections. Each connection consists of an aluminum probe surface that connects the micro-machined sensor through a backside bonding technique. A biologically inert substance is coated on the circuit leaving out the probe areas.
The array within the sensor consists of nearly 100 microelectrodes that monitor the response condition of the neurons. The array also includes a signal carrier, which is a bi-directional port that functions to transfer the neural signals from the neurons in a single line, all the while monitoring neuro-response. The microelectrode stimulates the neurons under a demultiplexer configuration of the array whilst monitoring the neural response in the visual cortex when the array is in multiplexer configuration. Each microelectrode is composed of two n-channel MOSFETs and a neural probe. These transistors are active components used for activating the microelectrode; however, the probes are passive components that enable neurons to interact with an electronic system.
The sensor is incorporated with a decoder that activates microelectrodes and transmits pulses of +5V to the row and column ports of the array. Finally, set and clock cycles were set in a way that directs the set signals to begin probe scanning.
Development of Electronic Sensors for Vision Prostheses
Advancements in sensor technology have enabled researchers to develop innovative visual prostheses to assist visually impaired people. Compared to the classical visual prostheses that utilize both a camera and a vision processor to capture and convert the images of a visual scene into waveforms, respectively, optical sensor prostheses instead solely relies on photodiode technology, rather than a camera, to obtain and convert visible light into electrical activity that will eventually be used to stimulate visual pathway neurons. Several clinical trials are currently being conducted in an effort to evaluate the efficacy of these types of optical sensor prostheses. Discussed below are some of the interesting novel approaches used in the development of visual implants.
Researchers working at the Boston Retinal Implant Project
have developed a retinal implant comprising power-and-data-receiving coils, an external video-capture unit, discrete power-supply components, and a 15-channel stimulator chip. A subretinal part of a pig was implanted with an array while the sclera was implanted with an encased electronics assembly. A serpentine electrode array stretches to the eye's superior temporal quadrant. Also, a transmitter is used for sending information about the image to the implanted device. The image is translated into biphasic current pulses using an application-specific integrated circuit.
Another experiment carried out by the Loudin J.D. et al (2011) at Stanford University uses photodiode circuits for producing high-resolution retinal prostheses. A data stream obtained from a video camera is processed by a pocket PC, and the resulting images are displayed on a micro-LCD which is illuminated by a 900-nm infrared light at intervals of 0.5-ms. Consequently, the images are pushed into the retina and received by photovoltaic pixels. The pulsed light is then transformed into pulsed biphasic electric current for carrying image information to diseased retinal tissue.
Afterward, Loudin examined the complex interaction between the electrode, photodiode, and light with theoretical measurements and calculations. Active photoconductive and passive photovoltaic circuits were investigated. The team of researchers observed that the sputtered iridium–oxide film (SIROF) electrodes differ from platinum electrodes in terms of charge injection levels and circuit behavior. The experiment concluded that photovoltaic and photoconductive systems are suitable for high-resolution optoelectronic retinal prostheses.
Another experiment by another group of researchers in 2009 involved developing a monitoring system for older people to recognize their actions, behavior and unusual patterns automatically by means of sensors and robotic systems for supporting their movement. The system developed used an omni-directional vision sensor and a fuzzy inference approach for detecting motions and behavior patterns. This approach was designed was helped by the values of center of gravity and the area of the obtained image, and frequency of the pattern appearance. Similarly, unusual patterns can be identified using a possibility distribution inference method.
An Overview of Retinal Prostheses Development
Worldwide, several research groups are looking to stimulate the retina artificially by using implantable devices for providing vision. Since Massachusetts’s Eye and Ear/MIT group, in collaboration with the North Carolina State group introduced their electrical retinal stimulator, several retinal prosthetic devices have undergone clinical trials. These include the Second Sight Medical Products, Inc.’s Argus II epiretinal and Retina Implant AG’s subretinal devices.
Clinical trials on the Argus II device, which is implanted epiretinal, demonstrated that this device successfully elicits phosphenes in all test subjects, improves their visual acuity, and enhances their orientation and mobility performance. The successful application of the Argus II in these early trials inspired the company to assess whether this retinal prosthesis could repair the vision loss of patients who have suffered from atrophic age-related macular degeneration (AMD).
Several other technology companies have found promising results on their retinal prostheses. For example, Bionic Vision and Bionic Vision Technologies, which are two Australia-based companies, have recently developed a suprachoroidal device that is polaced between the choroid and the sclera of the eye. Here in the eye, which is farther away from target neurons than other retinal prosthetic devices, Bionic Vision’s suprachoroidal device can be implanted in a minimally invasive manner, while simultaneously remaining effective at stimulating the retina when implanted. The clinical applications of the Bionic Vision device have successfully undergone two trials, during which phosphenes were successfully elicited light localization of up to 97.5% was achieved and improved visual acuity scores were recorded.
Conclusion
As sensor technology continues to advance, a vast number of prosthetic devices will continue to emerge to correct visual functionality in visually impaired individuals. Also, to the prosthetic devices discussed here, a number of other prostheses including both optic nerve and cortical prostheses have emerged. With further work in this area, it is inevitable that the future of optical sensor prostheses will provide sight restoration to thousands of people around the world.
Sources and Further Reading
- Loudin, J.D., Cogan, S. F., Mathieson, K., Sher, A., & Palanker, D. V. (2011). Photodiode Circuits for Retinal Prostheses. IEEE Transactions on Biomedical Circuits and Systems 5(5). DOI: 10.1109/TBCAS.2011.2144980.
- Kelly, S.K., Shire, D.B., Chen, J., Doyle, P., Gingerich, M.D., et al. (2011). A hermetic wireless subretinal neurostimulator for vision prostheses. IEEE Transactions on Biomedical Engineering 58(11); 3197–3205. DOI: 10.1109/TBME.2011.2165713.
- Schwiebert, L., Gupta, S.K.S., Auner, P.S.G., Abrams, G., Iezzi, R., & McAllister, P. (2002). A biomedical smart sensor for the visually impaired. Sensors, Proceedings of IEEE. 693-698. DOI:10.1109/ICSENS.2002.1037187.
- Seki, H. (2009). Fuzzy inference based non-daily behavior pattern detection for elderly people monitoring system. Conference Proceedings - IEEE Engineering in Medicine and Biology Society. 6187-6192. DOI: 10.1109/IEMBS.2009.5334614.
- Brandii, A., Luu, C. D., Guymer, R. H., & Ayton, L. N. (2016). Progress in the clinical development and utilization of vision prostheses: an update. Eye Brain 8; 15-25. DOI: 10.2147/EB.S70822.
This article was updated on 14th February, 2020.
Disclaimer: The views expressed here are those of the author expressed in their private capacity and do not necessarily represent the views of AZoM.com Limited T/A AZoNetwork the owner and operator of this website. This disclaimer forms part of the Terms and conditions of use of this website.