In this article, we explore how implantable sensors could offer more in-depth, personalized insights into health. Learn about the history behind health monitoring, the materials conventionally used in implantable sensors, and what the future may hold for this technology.
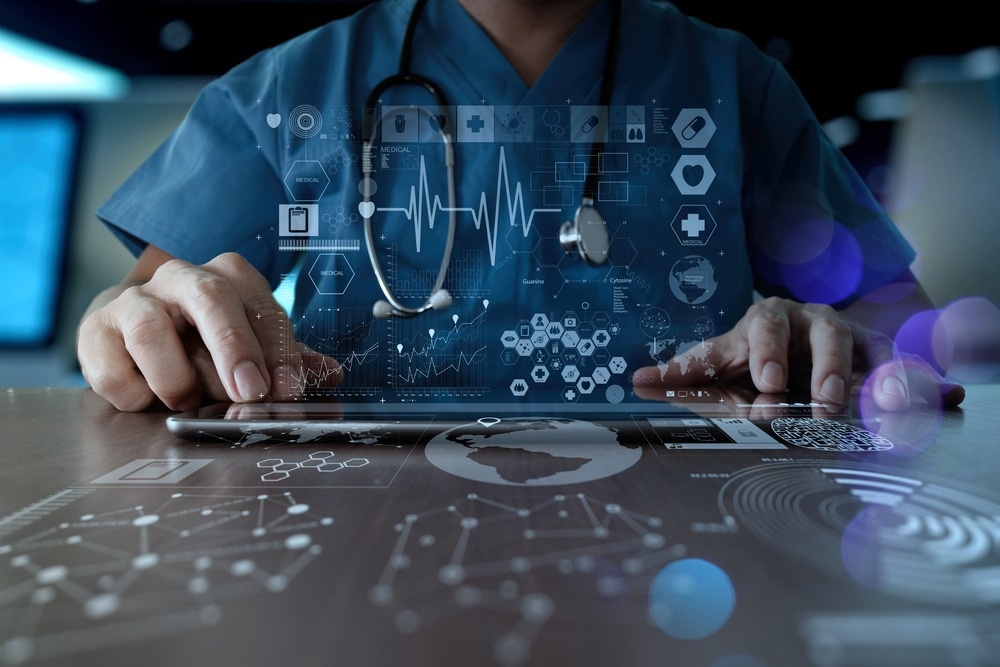
Image Credit: everything possible/Shutterstock.com
Technologies for Monitoring Health: A History
Devices intended for the monitoring of health have been developed for centuries, arguably millennia, as sound-amplifying devices intended for listening to the heart and lungs are referenced in the Ebers Papyrus, dated 5,000 BCE.
In 1625, Santorio of Venice wrote on the importance and methods of measurement of body temperature, and devised a way of recording pulse rate using a swinging pendulum.
The importance of monitoring patient breathing rate was then highlighted in 1852 by Ludwig Taube, and later that century in 1896 the world’s first blood pressure cuff (sphygmomanometer) was introduced, establishing the four vital signs: body temperature, pulse rate, breathing rate, and blood pressure.
Shortly after, Willem Einthoven earned a Nobel prize for his invention of the string galvanometer for the measurement of electrocardiograms.
By the 1950s and 60s, electronic technology had advanced to the point that these measurements could be performed simultaneously and continuously, and the cardiotachoscope and its modern equivalents came about.
The early pacemaker was also steadily developed over the course of several decades owing to contributions from numerous researchers and physicians. It was first noted in the British Medical Journal, 1889, that application of electrical impulses to the asystole heart caused ventricular contraction. By 1928, the first device was used to revive a stillborn infant following ten minutes of stimulation.
The first transcutaneous pacemakers were developed in the 1950’s, and in some cases, were required to be plugged directly into wall sockets or came with bulky batteries; one example was developed by Columbian doctor and engineer team Alberto Vejarano Laverde and Jorge Reynolds Pombo weighing 45 kg.
The creation of the earliest silicon transistors allowed significant miniaturization of electronics compared to prior vacuum tube technology and led to the production of more practical wearable pacemakers, as well as other health monitoring technologies. This article will discuss some of the recent innovations surrounding health monitoring, in particular implantable devices and the key technologies that make them feasible.
Types of Implantable Sensor
The most important property of any implantable monitor, sensor, or health-modulating device is biocompatibility, as it must be ensured that the body will tolerate the material on a long-term basis without significant degradation.
Inappropriate materials may cause both local and systemic damage through a variety of biochemical interactions. Copper, for example, has a high surface energy and is capable of leaching copper ions into surrounding biological media, both of which promote the generation of reactive oxygen species and cause local necrosis.
Some materials, such as nickel impurities, may be capable of eliciting an immune response, causing systemic inflammation and potentially cascading health issues. Inert and biocompatible polymers and some noble metals such as platinum make effective coating materials for implantable devices, though other potentially dangerous materials must still be used to construct the inner electronic components.
Ceramics and some 2D electronic materials, such as graphene, possess interesting properties that may be exploited in future implantable sensors: biocompatibility, flexibility, reduced size, and improved sensitivity.
2D electronic materials are of particular interest as biosensors as they pose an extremely large surface-to-volume ratio with highly customizable surface chemistry, allowing them to be modified with specific biochemical probes such as antibodies.
When a compatible antigen then bonds with the 2D sensor-bound antibody, a change in resistivity is experienced by the electrically conductive material, providing a quantitative measure of the presence of the molecule of interest.
The applications of this technology within implantable devices are obvious and wide, ranging from the continuous monitoring of blood glucose in diabetic patients to the early alert of pathogenic invasion.
The flexibility of 2D materials also lends them use as movement and pressure sensors, with immediate applications in the continuous detection of intraocular and intracranial pressure, which can be indicative of a number of diseases and serious health conditions.
Data Transmission and Power
Wireless power and data transmission are increasingly commonplace in modern electronics. Bluetooth and the Internet of Things are incorporated into most communication devices, and everyday items such as electric toothbrushes are charged electromagnetically.
Using this type of technology, the device and charger must be brought very closely together, hundreds of millimeters at most, and thus is not particularly useful for charging deeply implanted devices.
Further, both power and data losses incurred by transmission through human tissue must be overcome. Non-radiative and radiative mid-field and radiative far-field technologies can transfer power deep inside the human body; however, these powering schemes suffer from low power transfer efficiency, which limits the applications to ultra-low-power electronics, and the high frequencies involved may be damaging to human tissues long-term.
The aforementioned flexibility of 2D materials may potentially be used to power implantable devices, where the kinetic energy imparted by day-to-day movement is stored in capacitors that can then slowly feed energy to the device.
Conclusions
Amongst the most exciting prospects of fully biocompatible biosensors with convenient or in-built power supply and data sharing is their broad adaptability, particularly when incorporated with the sensing capabilities of 2D electronic materials.
Biosensors customized specifically to the sensing of disease biomarkers in high-risk patients would provide early warning of changes in condition, such as cancer entering the stage of metastasis, genetic diseases emerging, or monitoring the response to a therapeutic treatment.
With the improving ability to manufacture complex and biocompatible microfluidic components, highly attuned sensing devices could be directly incorporated into implanted medicine administration apparatus, allowing signs of oncoming medical emergencies to be detected and treated early.
References and Further Reading
Liu, G. et al. (2023). Biocompatible Material-Based Flexible Biosensors: From Materials Design to Wearable/Implantable Devices and Integrated Sensing Systems. Small, 19(27). doi.org/10.1002/smll.202207879
Gray, M. E., et al. (2019). Biocompatibility of common implantable sensor materials in a tumor xenograft model. Journal of Biomedical Materials Research Part B: Applied Biomaterials, 107(5), pp. 1620–1633. doi.org/10.1002/jbm.b.34254
Khan, S. R., et al. (2020). Wireless Power Transfer Techniques for Implantable Medical Devices: A Review. Sensors, 20(12), p. 3487. doi.org/10.3390/s20123487
Du, Y., et al. (2020). Research Progress of Graphene-Based Materials on Flexible Supercapacitors. Coatings, 10(9), p. 892. doi.org/10.3390/coatings10090892
Disclaimer: The views expressed here are those of the author expressed in their private capacity and do not necessarily represent the views of AZoM.com Limited T/A AZoNetwork the owner and operator of this website. This disclaimer forms part of the Terms and conditions of use of this website.