By Ankit SinghReviewed by Susha Cheriyedath, M.Sc.Nov 22 2024
Integrated microfluidic sensors have revolutionized diagnostics by combining precision engineering, fluid dynamics, and biochemical analysis within compact, cost-effective devices. These lab-on-a-chip (LOC) technologies enable real-time monitoring, rapid analysis, and high sensitivity, making them indispensable for point-of-care (POC) diagnostics.
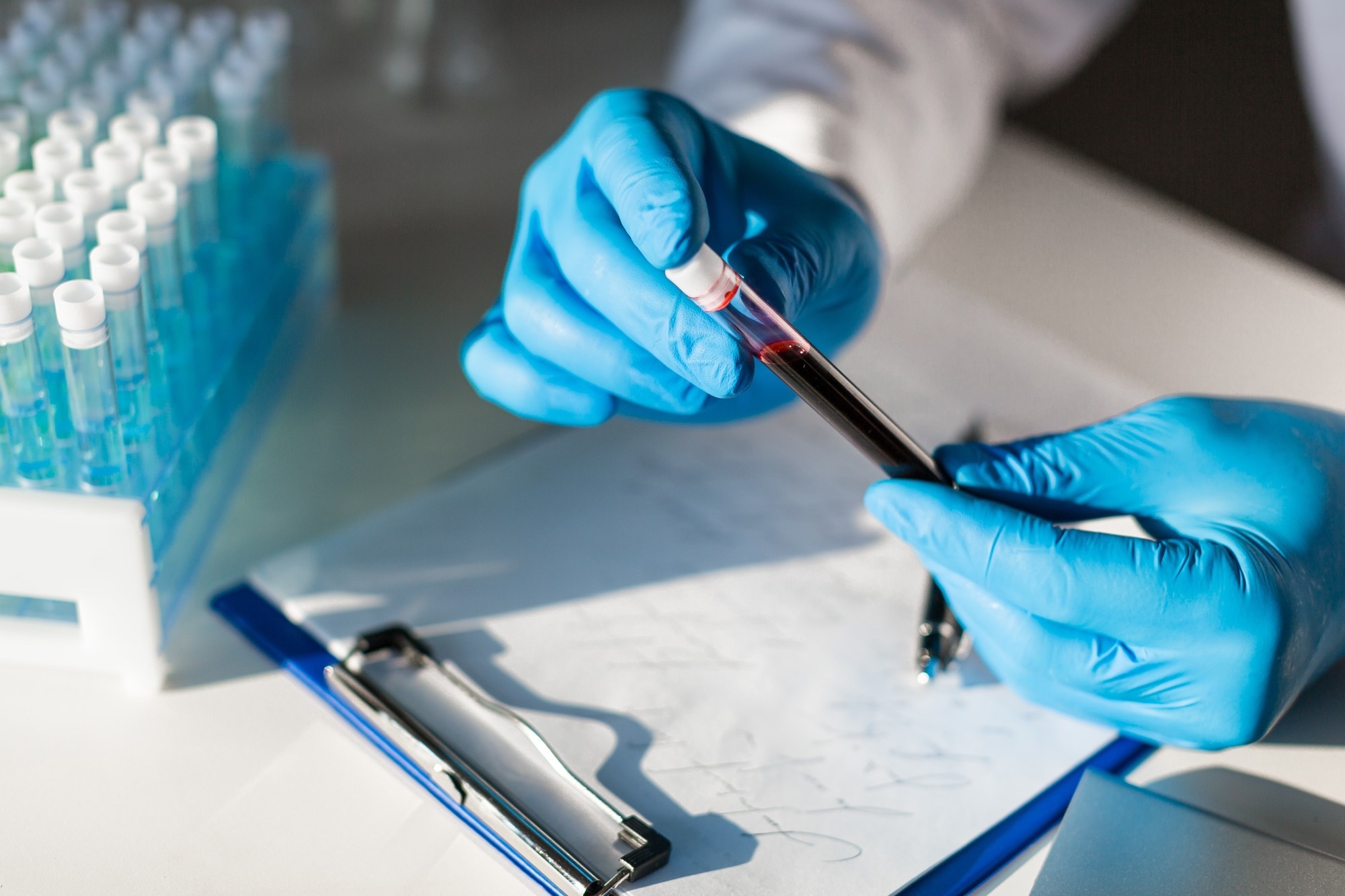
Image Credit: Green Elk/Shutterstock.com
From healthcare to environmental monitoring, their versatility continues to expand the frontiers of modern science. This article looks at the concept, applications, recent advancements, challenges, and future prospects of integrated microfluidic sensors, shedding light on their role in revolutionizing POC diagnostics.
What Are Integrated Microfluidic Sensors?
Integrated microfluidic sensors are miniaturized analytical devices designed to perform multiple laboratory functions on a single chip. They incorporate microfluidic channels, reservoirs, and detectors, enabling precise manipulation of tiny fluid volumes (nanoliters to microliters). Their integration into LOC systems makes complex diagnostic workflows portable, faster, and user-friendly.
Companies like Abbott Laboratories, Fluidigm, and Dolomite Microfluidics have been at the forefront of designing innovative integrated microfluidic systems. These systems enhance laboratory efficiency by miniaturizing complex processes onto a single chip.
For instance, Fluidigm is known for its high-throughput platforms, such as the Biomark HD™, which allows simultaneous gene expression analysis across numerous samples. Similarly, Abbott Laboratories has made strides in point-of-care diagnostics using microfluidic technology to enable rapid and accurate testing. Dolomite Microfluidics excels in providing custom microfluidic tools tailored to applications ranging from drug discovery to environmental monitoring, offering precise control over fluid dynamics—crucial for a variety of analytical tasks. Collectively, these companies are at the forefront of microfluidic innovation, driving progress in genomics, diagnostics, and biomedical research.1,2
What is Microfluidics?
Breaking Down the Chip: The Inner Workings of Microfluidic Sensors
Integrated microfluidic sensors rely on a carefully coordinated array of components to perform precise biochemical analyses. At the heart of these sensors are microfluidic channels, which are tiny pathways etched or molded into the chip. These channels are designed to control fluid movement using principles of laminar flow, allowing for the precise handling of samples and reagents.
The nanoscale dimensions of these channels introduce unique fluid dynamics, where phenomena such as surface tension and electrokinetic effects become significant. Understanding these behaviors is crucial for optimizing the performance of microfluidic systems, as they can dramatically influence reaction kinetics and transport mechanisms.
Sample injection systems play a critical role in ensuring the consistent introduction of biological samples into the microfluidic environment. Often automated, these systems are designed to minimize variability in sample volume and timing, which is essential for reproducibility and accuracy in analytical results. The precision of sample injection is particularly important when dealing with low-concentration analytes or when performing assays that require stringent control over reaction conditions.
Detection modules within integrated microfluidic sensors utilize various technologies to measure biochemical interactions. Electrochemical sensors detect changes in electrical properties resulting from biochemical reactions, making them highly sensitive for measuring metabolites like glucose or lactate. Optical detectors employ fluorescence or absorbance techniques to monitor molecular interactions, often incorporating on-chip light sources such as LEDs for miniaturized spectrophotometry.
Additionally, mass-based sensors, including piezoelectric and cantilever devices, detect mass changes associated with biomolecular binding events. Each detection method presents unique challenges, such as maintaining a high signal-to-noise ratio in miniaturized systems and developing advanced signal processing algorithms to accurately interpret the data.
Actuators and valves are crucial for regulating the flow and mixing of reagents within microfluidic devices. Pneumatic valves or electroosmotic pumps provide precise flow control, enabling complex assays that require specific timing and mixing protocols. The design and integration of these components must consider not only fluid mechanics but also material properties that can affect chemical reactions within the microchannels.
The integration of electronics with microfluidic sensors further enhances their functionality. Signal processors and wireless communication modules enable real-time data transmission to external devices, such as smartphones or cloud-based systems. This connectivity allows for remote monitoring and analysis, making integrated microfluidic sensors particularly valuable in point-of-care diagnostics and personalized medicine.
Together, these components work in unison to facilitate multi-step diagnostic assays. A biological sample is introduced into the chip, where it is manipulated within microfluidic chambers that allow for controlled biochemical reactions. Detectors measure the outcomes of these reactions, which are then processed into actionable data using integrated electronics. This streamlined operation not only enhances efficiency but also broadens the applicability of integrated microfluidic sensors across various fields, including genomics, proteomics, environmental monitoring, and clinical diagnostics.1,2
Learn more about the fabrication techniques that power integrated microfluidic sensors and lab-on-a-chip technologies.
Real-World Applications of Integrated Microfluidic Sensors
Integrated microfluidic sensors have found applications across a broad spectrum of diagnostic and monitoring scenarios. Their versatility and portability are driving their adoption in various settings:
- Infectious disease diagnostics
- Cancer diagnostics
- Chronic disease management
- Neonatal and prenatal care
- Environmental and food safety monitoring
- Drug development
In infectious disease diagnostics, these sensors have proven especially useful during the COVID-19 pandemic. They have enabled rapid point-of-care testing through real-time RT-PCR and antigen detection, facilitating faster decision-making in containment and treatment strategies. For diseases like tuberculosis, portable lab-on-a-chip devices have improved screening in underserved areas by efficiently processing sputum samples.3
Cancer diagnostics is another area where microfluidic platforms are making significant strides. These devices can analyze various cancer biomarkers, including circulating tumor cells (CTCs), circulating tumor DNA, and proteins. For instance, a microfluidic platform developed by Wang and colleagues can simultaneously detect multiple tumor markers such as CEA, AFP, and CA-125 using a chemiluminescence immunoassay-based paper analytical device (μPAD). This technology offers a non-invasive alternative to traditional biopsies and aids in early-stage cancer detection.4
In chronic disease management, integrated microfluidic sensors have simplified the continuous monitoring of physiological parameters. For example, advanced sensors can measure glucose levels in real-time for diabetic patients, ensuring timely intervention. Similarly, in cardiac health, these sensors can be employed to analyze biomarkers like troponin to diagnose acute myocardial infarction, significantly reducing the diagnostic window.3
In neonatal and prenatal care, quick and accurate testing is vital for ensuring maternal and infant health. For instance, newborn screening now allows healthcare providers to detect metabolic disorders or genetic conditions within hours of birth, facilitating early intervention and improved long-term outcomes. Similarly, prenatal diagnostics use samples of amniotic fluid or maternal blood to identify chromosomal abnormalities, such as Down syndrome, providing parents and healthcare teams with critical information for making informed decisions during pregnancy.5
In pharmaceutical research, microfluidic platforms have become indispensable tools for drug screening and toxicity studies. By offering cost-effective and efficient methods, these technologies streamline the drug development process. Microfluidic systems can replicate human physiological conditions more effectively than traditional methods, providing researchers with accurate predictions of drug behavior. This capability not only accelerates the timeline for new drug development but also reduces costs, making these platforms essential for advancing modern pharmaceutical science.7
Beyond healthcare, microfluidic technologies also play a crucial role in detecting contaminants in water, air, and food, which is essential for ensuring public safety. For example, water quality testing using microfluidic chips can detect heavy metals or microbial contamination in real-time, allowing immediate action to prevent health risks. Similarly, microfluidic devices used in food safety can screen for pathogens like Salmonella or E. coli, reducing the risk of outbreaks and enhancing consumer confidence in food products.6
Challenges in Scaling Microfluidic Devices
While integrated microfluidic sensors offer remarkable potential, several hurdles limit their full-scale adoption and impact.
- Fabrication Complexity: Producing high-performance devices at scale, particularly those with multi-material designs, requires advanced and resource-intensive manufacturing techniques.1
- Cost Constraints: While microfluidic chips are cost-effective in research, high initial costs for commercial development and deployment can hinder widespread adoption.1
- Sample Variability: Biological sample heterogeneity poses challenges in achieving consistent results, especially for complex assays.1
- Regulatory Hurdles: Lengthy and costly approval processes for diagnostic devices delay commercialization and increase time-to-market.1
The Latest in Integrated Microfluidic Sensor Research
Recent advancements in integrated microfluidic sensors have pushed the boundaries of their functionality and accessibility. In a recent study published in ACS Sensors, scientists developed a 3D-printed flexible wearable health monitor for non-invasive, continuous sweat analysis.
Fabricated through a one-step process, the device features self-supporting microfluidic channels and integrates novel single-atom catalyst-based bioassays. This design eliminates contamination and evaporation issues while improving manufacturing efficiency via a pick-and-place strategy for bioassay integration. The monitor accurately measures sweat rate and biomarkers like glucose, lactate, and uric acid. A feasibility study on human skin demonstrated its functionality, offering a promising tool for real-time, reliable health monitoring during physical activity.8
Another breakthrough study published in Fundamental Research introduced an artificial intelligence (AI)-aided on-chip approach to improve PCR testing efficiency for SARS-CoV-2 detection. By integrating deep learning with microfluidic paper-based analytical devices (µPADs), the system accelerates diagnostics by forecasting results in just 13 cycles, reducing test time by 67.5 %.
The µPADs, compatible with conventional PCR instruments, utilize various kinds of deep neural networks [Recurrent Neural Network (RNN), Long Short-Term Memory (LSTM), and Gated Recurrent Unit (GRU)], with GRU proving the most effective. This approach also enables dynamic evaluation of critical cycles, allowing real-time quantitative analysis and accurate predictions of PCR trends and outcomes. The method is universally adaptable, offering innovative cross-disciplinary insights for enhancing diagnostic accuracy and efficiency across various research fields.9
Future Prospects and Conclusion
Integrated microfluidic sensors hold immense promise for the future, with innovations likely to enhance their performance and accessibility. Advances in nanotechnology, AI, and machine learning are expected to further refine sensor sensitivity and data analysis capabilities.
Emerging manufacturing innovations, such as high-resolution 3D printing and roll-to-roll fabrication, are not just lowering production costs—they are redefining scalability. These techniques make it feasible to produce microfluidic devices at volumes necessary for mass adoption, opening the door to widespread availability. Furthermore, combining microfluidic systems with wearable devices and IoT platforms could shift healthcare toward proactive models, where continuous health monitoring and early interventions become the norm, reducing the burden on reactive medical systems.
Despite their immense potential, the journey toward widespread adoption is not without challenges. Regulatory approval processes remain a significant hurdle, often slowing down commercialization. To address this, collaboration between academia, industry leaders, and regulatory bodies will be critical. Streamlining approval pathways and creating standards for equitable distribution will ensure these technologies reach underserved regions, amplifying their global impact.
References and Further Reading
- Buttkewitz, M. A. et al. (2023). Sensor integration into microfluidic systems: trends and challenges. Current Opinion in Biotechnology, 83, 102978. DOI:10.1016/j.copbio.2023.102978. https://www.sciencedirect.com/science/article/pii/S0958166923000885
- Mak, W. C. (2022). Microfluidic Biosensors. Elsevier. DOI:10.1016/C2019-0-05276-4. https://www.sciencedirect.com/book/9780128238462/microfluidic-biosensors
- Behera, P. P. et al. (2023). Integrated microfluidic devices for point-of-care detection of bio-analytes and disease. Sensors & Diagnostics. DOI:10.1039/d3sd00170a. https://pubs.rsc.org/en/content/articlehtml/2023/sd/d3sd00170a
- Sierra, J. et al. (2020). Sensor-Integrated Microfluidic Approaches for Liquid Biopsies Applications in Early Detection of Cancer. Sensors, 20(5), 1317. DOI:10.3390/s20051317. https://www.mdpi.com/1424-8220/20/5/1317
- Kim, J. et al. (2023). Skin-interfaced wireless biosensors for perinatal and paediatric health. Nat Rev Bioeng 1, 631–647. DOI:10.1038/s44222-023-00090-0. https://www.nature.com/articles/s44222-023-00090-0
- Alhalaili, B. et al. (2022). Microfluidic Devices and Microfluidics-Integrated Electrochemical and Optical (Bio)Sensors for Pollution Analysis: A Review. Sustainability, 14(19), 12844. DOI:10.3390/su141912844. https://www.mdpi.com/2071-1050/14/19/12844
- Mao, K. et al. (2020). Paper-based microfluidics for rapid diagnostics and drug delivery. Journal of Controlled Release, 322, 187–199. DOI:10.1016/j.jconrel.2020.03.010. https://www.sciencedirect.com/science/article/abs/pii/S0168365920301620
- Chen, C. et al. (2024). 3D-Printed Flexible Microfluidic Health Monitor for In Situ Sweat Analysis and Biomarker Detection. ACS Sensors. DOI:10.1021/acssensors.4c00528. https://pubs.acs.org/doi/full/10.1021/acssensors.4c00528
- Sun, H. et al. (2021). AI-aided on-chip nucleic acid assay for smart diagnosis of infectious disease. Fundamental Research. DOI:10.1016/j.fmre.2021.12.005. https://www.sciencedirect.com/science/article/pii/S2667325821003095
Disclaimer: The views expressed here are those of the author expressed in their private capacity and do not necessarily represent the views of AZoM.com Limited T/A AZoNetwork the owner and operator of this website. This disclaimer forms part of the Terms and conditions of use of this website.