Making use of ultrasound to analyze the biomechanical properties of tissues could assist in detecting and controlling pathophysiological conditions, tracking the progress of lesions, and assessing the advancement of rehabilitation.
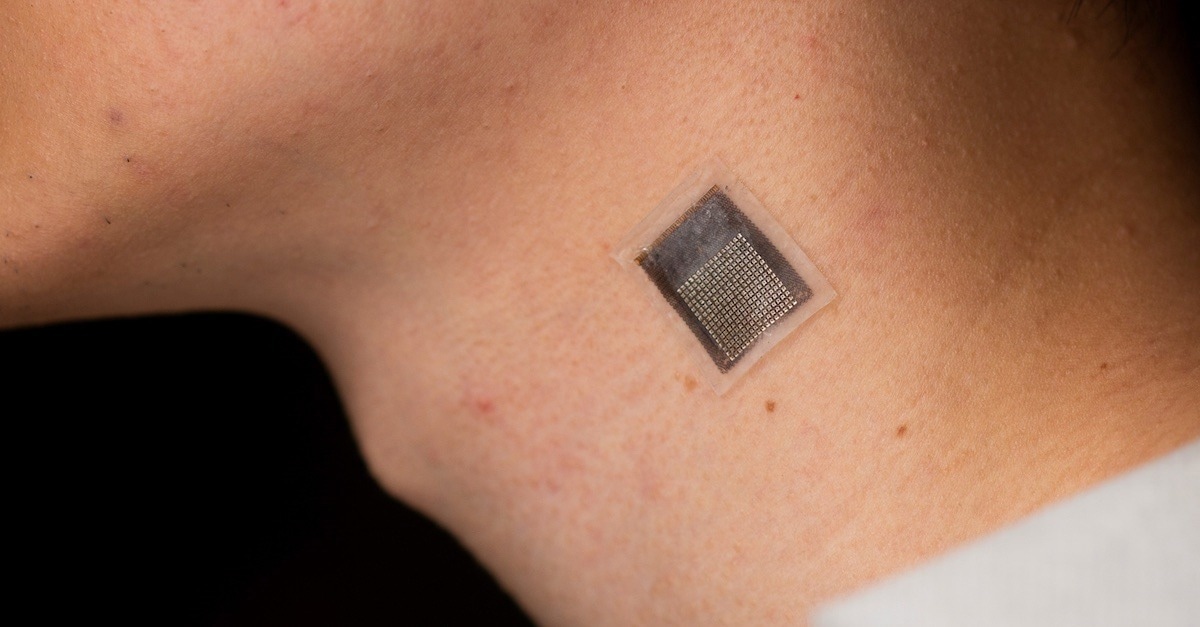
Optical images of the devices when twisted and stretched, showing its mechanical compliance and robustness, and conforming to the lateral shoulder joint and neck for tissue stiffness monitoring. Image Credit: Jacobs School of Engineering, University of California San Diego
An engineers’ group at the University of California San Diego has come up with a stretchable ultrasonic array that streamlines serial, non-invasive, three-dimensional imaging of tissues as deep as 4 cm below the surface of human skin at a spatial resolution of 0.5 mm. This new technique offers a non-invasive and longer-term alternative to present techniques, with enhanced penetration depth.
The study appears from the lab of Sheng Xu, a professor of nanoengineering at UC San Diego Jacobs School of Engineering and the corresponding author of the study.
We invented a wearable device that can frequently evaluate the stiffness of human tissue. In particular, we integrated an array of ultrasound elements into a soft elastomer matrix and used wavy serpentine stretchable electrodes to connect these elements, enabling the device to conform to human skin for serial assessment of tissue stiffness.
Hongjie Hu, Study Co-Author and Postdoctoral Researcher in the Xu Group, University of California-San Diego
The elastography monitoring system has the potential to offer serial, non-invasive, and three-dimensional mapping of mechanical properties available for deep tissues. This has numerous main applications:
- In the field of medical research, serial data on pathological tissues has the potential to offer vital data on the advance of diseases like cancer, which generally makes cells harden.
- Monitoring muscles, tendons, and ligaments could assist diagnose and treat sports injuries.
- Present treatments available for cardiovascular and liver illnesses, together with a few chemotherapy agents, might impact tissue stiffness. Constant elastography could help evaluate the efficacy and delivery of such medications. This may aid in making novel treatments.
Besides tracking cancerous tissues, this technology could also be employed in other scenarios:
- Tracking of cirrhosis and fibrosis of the liver. By making use of this technology to assess the severity of liver fibrosis, it is possible for medical professionals to precisely track the advance of the disease and identify the most suitable course of treatment.
- Evaluating musculoskeletal disorders like tennis elbow, tendonitis, and carpal tunnel syndrome. By monitoring variations in tissue stiffness, this technology has the potential to offer useful knowledge into the progression of these conditions. This enables doctors to come up with individualized treatment plans for their patients.
- Diagnosis and monitoring for myocardial ischemia. By monitoring arterial wall elasticity, it becomes easy for doctors to determine early signs of the condition and make early interventions to avoid additional damage.
The Xu Lab: A Leader in Wearable Ultrasound
Over the past few decades, and as a result of the technological advances and the hard work of clinicians, ultrasound has received a continuing wave of interest, and the Xu lab is frequently referred in the first breath as an early and lasting leader in the field, specifically in wearable ultrasound.
The laboratory took devices that were portable and stationary and made them wearable and stretchable, driving a shift throughout the landscape of healthcare monitoring.
Wearable ultrasound patches achieve the detection function of conventional ultrasound and also break the restrictions of conventional ultrasound technology, like one-time testing, testing only within hospitals, and also the need for staff operation.
Hu stated, “This allows patients to continuously monitor their health status anytime, anywhere.”
This could aid in reducing fatalities and misdiagnoses, as well as considerably lower costs by offering a non-invasive and affordable alternative to conventional diagnostic procedures.
This new wave of wearable ultrasound technology is driving a transformation in the healthcare monitoring field, improving patient outcomes, reducing healthcare costs, and promoting the widespread adoption of point-of-care diagnosis. As this technology continues to develop, it is likely that we will see even more significant advances in the field of medical imaging and healthcare monitoring.
Yuxiang Ma, Study Co-Author and Visiting Student in the Xu Group, University of California-San Diego
How it Works
The array adapts to the human skin and acoustically pairs with it, thereby enabling precise elastographic imaging confirmed with magnetic resonance elastography.
As far as testing is concerned, the device was utilized to map three-dimensional distributions of Youngs’ modulus of tissues ex vivo to detect microstructural damage caused in the muscles of volunteers before the onset of soreness and track the dynamic recovery process of muscle injuries at the time of physiotherapy.
We choose 3 MHz as the center frequency for the ultrasound transducer. Since the higher the center frequency, the higher the spatial resolution, but the stronger the attenuation of the ultrasound wave in tissues, 3 MHz satisfies the requirements for both high spatial resolution and superb tissue penetration.
Hongjie Hu, Study Co-Author and Postdoctoral Researcher in the Xu Group, University of California-San Diego
The device comprises a 16 by 16 array. Every element is made of a 1-3 composite element and a backing layer created from a silver-epoxy composite developed to absorb surplus vibration, widening the bandwidth and enhancing axial resolution.
“We choose a pitch - the distance between the centers of two adjacent elements - of 800 μm, which is adequate for producing high-quality images, minimizing interference from neighboring elements, and giving the entire device its good stretchability,” stated Xiaoxiang Gao, another postdoctoral researcher in the group.
Specifications:
- Dimensions: nearly 23 mm × 20 mm × 0.8 mm
- Biaxial stretchability: 40%.
- Penetration depth: greater than 4 cm
- Highest signal-to-noise ratio: 28.4 dB
- Spatial resolution: 0.5 mm
- Contrast resolution: 1.74 dB
Overcoming Challenges
In the sample, such technology should record the motion of scattering particles by ultrasound wave and assess their displacement fields depending on a normalized cross-correlation algorithm. The size of scattering particles is very compact, leading to a weak reflected signal. Capturing such faint signals requires sensitive technology.
Present fabrication techniques include high-temperature bonding that could result in serious and irreversible thermal damage to the epoxy in the piezoelectric materials. Consequently, the sensitivity of the transducer element degrades considerably.
Hu added, “To address those challenges, we developed a low-temperature bonding approach. We replaced the solder paste with conductive epoxy, which allows the bonding to be completed at room temperature without causing any damage to the element.”
“In addition, we replaced the single plane wave transmission mode with coherent plane-wave compounding mode, which provides more energy to boost the signal intensity throughout the entire sample. Using these strategies, we improve the sensitivity of the device to make it perform well in capturing those weak signals from scattering particles.”
Next Steps
“A layer of elastomer with known modulus, the so-called calibration layer, can be installed on our device to further obtain quantitative, absolute values of tissues' moduli. This approach would allow us to obtain more complete information about tissues' mechanical properties, thus further improving the diagnostic capabilities of the ultrasonic devices,” stated Dawei Song, a postdoctoral researcher at the University of Pennsylvania and study coauthor.
Besides, advanced lithography, pick-and-place, and dicing methods could be adopted to additionally improve the array design and fabrication. This can help reduce the pitch and extend the aperture to obtain a higher spatial resolution and a wider sonographic window.
Gao stated, “It would be easier to explore opportunities working with physicians, pursuing potential practical applications in clinics. Our device shows great potential in close monitoring of high-risk groups, enabling timely interventions at urgent moments.”
This study was supported in parts by the Air Force Research Laboratory (AFRL) under agreement number FA8650-18-2-5402, the National Institutes of Health grants 1R21EB025521-01, 1R21EB027303-01A1, and 3R21EB027303-02S1, and 1R01EB033464-01, and the Center for Wearable Sensors at the UC San Diego.
Journal Reference
Hu, H., et al. (2023) Stretchable ultrasonic arrays for the three-dimensional mapping of the modulus of deep tissue. Nature Biomedical Engineering. https://doi.org/10.1038/s41551-023-01038-w.