Advancements within the field of wireless technology, wearable electronics, smart materials, and functional fibers have enhanced the mechanical, optical, and electrical capabilities of biosensing systems. While the utility of these sensors has been widely accepted for bench-top diagnostic processes, their incorporation into wearable devices has remained limited.
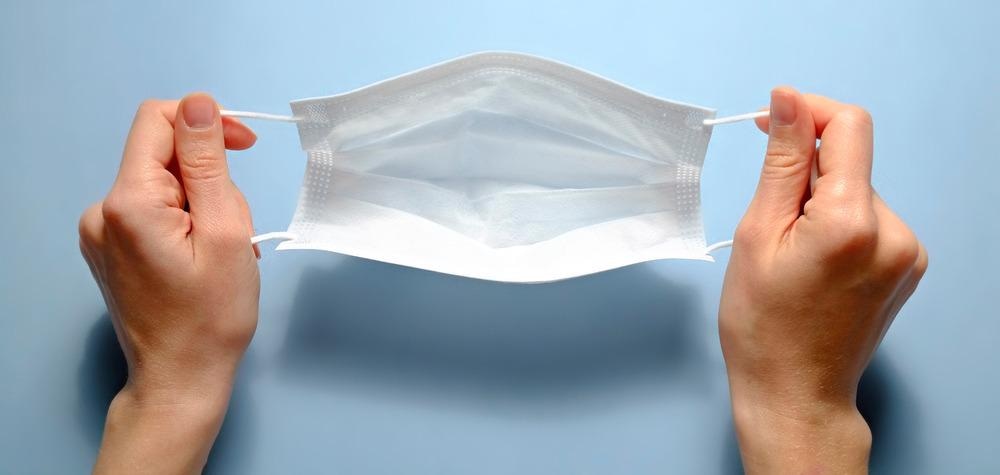
Researchers have developed sensors that can be placed inside face masks to detect viruses. Image Credit: evrymmnt/Shutterstock.com
This is largely due to the difficulty that scientists have encountered when attempting to retain the viability of the living organisms within these devices. More specifically, living cells require nutrients, a regulated environment with specific temperature and gas requirements, as well as waste extraction mechanisms. The use of genetically engineered cells can also pose biocontainment and/or biohazard risks, particularly when these organisms are integrated into garments available for consumer purchase.
Cell-free synthetic biology reactions are self-contained chemical systems that contain all the biomolecular components that are needed to perform transcription and translation at the cellular level. In addition, these systems can be lyophilized, or freeze-dried, expanding the ability to store and widely distribute these devices without specialized environmental or biocontainment requirements.
Designing a Wearable Sensor for Virus Detection
One of the key advantages associated with cell-free synthetic biological systems is that genetically engineered circuits can be added to these freeze-dried cell-free (FDCF) reactions for their activation. One recent development of an FDCF genetic circuit was discussed in a Nature Biotechnology paper, in which the researchers combined the circuits into flexible and textile substrates that can detect metabolites, chemicals, and pathogenic nucleic acid signatures.
To construct these systems, colorimetric genetic circuits were embedded into cellulose substrates that were surrounded by a containment assembly comprised of flexible elastomers. The genetic circuits were layered on top of one another to form reaction chambers that are directly connected to the top sample portals.
In the outermost layer of the device, portals were installed so that any fluid that is splashed into these areas of the device is rapidly absorbed into the hydrophilic paper networks that comprise the reaction chambers through capillary action.
To prevent the unwanted release of the sample, hydrophobic chamber walls were incorporated into these portals. Once the sample enters the circuit, lacZ β-galactosidase is hydrolyzed to chlorophenol red-β-D-galactopyranoside (CPRG). This chemical reaction will cause the color to change from yellow to purple, depending upon whether the target of interest is present within the sample.
Evaluating the Wearables’ Biosensing Capabilities
To test the sensitivity of these wearable devices, the researchers used four different synthetic biology biosensors with lacZ as the output. Taken together, all four of the wFDCF sensors tested were found to exhibit visible changes within 40 to 60 minutes after exposure to the target molecules or inducer.
Once the detection capabilities of the circuits were confirmed, the researchers sought to evaluate how the FDCF sensors functioned when incorporated into wearable fabrics and individual threads. After screening over 100 different fabrics, the researchers found that a fabric comprised of 85% polyester and 15% polyamide was chosen as the main immobilization substrate.
Both fluorescence and luminescence tests were performed to verify the functionality of this textile platform. For the fluorescence demonstrations, the wFDCF textiles would react with blue light at a wavelength of 447 nanometers (nm). Any light that was emitted from the system was then collected by a set of polymeric optic fibers (POFs), which then exited the fabric wave until reaching the optical sensor of the wearable device. Any signals that were emitted from the device were filtered and processed to provide fluorescence images of the POF bundle-ends.
For the luminescence demonstrations, all POF bundles were treated as signal emission sensors and did not require prior illumination of the sample. The luminescence demonstrations were conducted using a nanoluciferase operon that was downstream of two toehold switches for the RNA material of both HIV and Borrelia burgdorferi, the latter of which is the bacterium responsible for Lyme disease.
CRISPR-Based wFDCF Sensors
As compared to conventional synthetic biology sensors, the researchers sought to utilize Clustered Regularly Interspaced Short Palindromic Repeats (CRISPR) and CRISPR-associated (Cas) enzymes. These CRISPR-based approaches were utilized because of their numerous advantages, some of which include high sensitivity, rapid output, freeze-drying compatibility, ease of programmability, and simple base-pair resolution.
To this end, CRISPR-based specific high-sensitivity enzymatic reporter unlocking (SHERLOCK) sensors were incorporated into the fluorescence wFDCD platform. To evaluate the utility of this engineered system for wearable applications, the researchers tested the sensor against common resistance markers in Staphylococcus aureus.
Face Masks to Detect SARS-CoV-2
The researchers also explored whether their wFDCF system could be incorporated into face masks for the detection of SARS-CoV-2. Four modular components were included in the sensor, which was located on the inner side of the mask that is in direct contact with the wearer’s face.
These four components included:
- Reservoir for hydration
- Large surface area collection sample pad
- Wax-patterned microfluidic paper-based analytical device (µPAD)
- Lateral flow assay (LFA) strip
Breathing, talking, and/or coughing will lead to the release of respiratory droplets onto the sensor within the mask. When fluid or viral particles within the droplets reach the sample collection pad, capillary action brings the sample to the µPAD. Within the µPAD is an arrangement of lyophilized lysis and detection components, which is where several reactions take place until ultimately determining whether SARS-CoV-2 is present in the wearer’s respiratory droplets.
The current face mask sensor requires about 1.5 hours to collect and analyze respiratory samples for the presence of SARS-CoV-2. With a limit of detection of 500 copies, this sensor matches that of the reverse-transcriptase polymerase chain reaction (RT-PCR) assays that have been validated for clinical use by the World Health Organization. Importantly, the sensor was designed to specifically detect SARS-CoV-2 and will not cross-react with any other viral RNA material from other commonly circulating human coronavirus strains.
Conclusion
There are several advantages associated with the wFDCF sensor described here, particularly when applied to the testing of SARS-CoV-2 for face mask sensors. For example, the face mask sensor does not require any laboratory equipment or trained professionals to conduct this experiment. Furthermore, the sensor has no power source and instead operates autonomously, all while remaining stable for extended periods of time.
References and Further Reading
Synthetic biology [Online]. Available from: https://www.genome.gov/about-genomics/policy-issues/Synthetic-Biology
Hicks, M., Backmann, T. T., & Wang, B. (2019). Synthetic Biology Enables Programmable Cell-Based Biosensors. ChemPhysChem 21(2); 132-144. doi:10.1002/cphc.201900739
Nguyen, P. Q., Soenksen, L. R., Donghua, N. M., et al. (2021). Wearable materials with embedded synthetic biology sensors for biomolecule detection. Nature Biotechnology. doi:10.1038/241587-021-00950-3
Disclaimer: The views expressed here are those of the author expressed in their private capacity and do not necessarily represent the views of AZoM.com Limited T/A AZoNetwork the owner and operator of this website. This disclaimer forms part of the Terms and conditions of use of this website.