Oct 21 2012
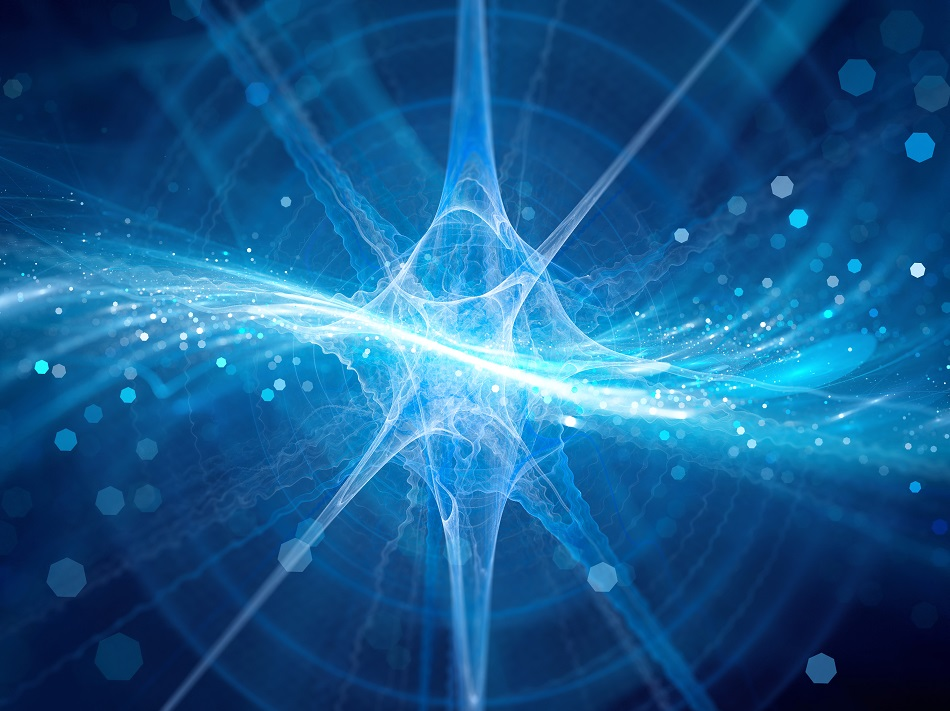
Image Credit: sakkmesterke/Shutterstock.com
A substance that emits low-energy photons when hit by a high-energy charged particle is known as a scintillator. Interaction of the gamma rays with scintillator crystals results in the generation of charged particles in the crystals. This charged energy is then emitted by the crystals in the form of photons. Scintillator crystals emit low-energy photons, collected by photomultiplier tubes.
High-energy electrons or positrons are produced when gamma rays passing through matter undergo one of the following processes:
• Pair production
• Photoabsorption
• Compton scattering
These positrons/electrons serve as charged particles in the scintillator. Thus, the gamma-ray’s energy is determined by the energy collected by photomultiplier tubes.
Scintillators are made up of different materials based on their applications. Inorganic materials like cesium iodide (CsI) or sodium iodide (NaI) make the scintillators used for gamma-ray detection. An impurity known as “activator” – sodium or thallium – is added to these materials for detecting gamma rays. Moreover, inorganic scintillators are used during space-based missions for observing cosmic gamma rays. The following video demonstrates liquid scintillation and the advantages and disadvantages of this process.
radiation detection with a scintillation counter / NaI(Tl) - sensitivity / efficiency
Inorganic Scintillators
Inorganic scintillators are crystals made of alkali metals, particularly alkali iodides, in addition to a small amount of impurity. The mechanism behind the inorganic scintillation process is discussed below:
The process of luminescence from the inorganic scintillators can be explained using the energy bands of the crystal.
Energy states in a crystal can be created between the conduction and valence bands in an atom, besides the exciton band due to activator atoms like thallium or imperfections in the crystal. The activator atoms can be observed in excited and ground states. However, photons are emitted in the order of 10-8s when the impurity atom is transferred from an excited, to a ground state. Scintillation occurs if the emitted photon has a wavelength in the visible region of the electromagnetic spectrum.
Thus, the occurrence of the following events results in scintillation:
- Ionization radiation is transmitted through the crystal
- Electrons are excited to the conduction band
- Holes are formed in the valence band
- Excitons are created
- Activation centers are activated to the excited states by absorption of excitons, holes, and electrons
- Photons are emitted after de-excitation
Organic Scintillators
The scintillation in organic scintillators is due to transitions between energy levels in a single molecule. These scintillators avoid the need for a regular crystal lattice structure. In organic scintillator molecules, the energy spacing the vibrational modes is 0.15eV, while between the levels S0 and S1 are 3eV. Excitation of molecules from a ground state to the higher states occurs when energy is stored in the scintillator by a charged particle. Phosphorescence is emitted when the molecule is transferred from the S1 to the T1 state.
A bulk solvent is added to the organic scintillant at small concentrations and the mixture is exposed to ionizing radiation. The process of transfer of solvent absorbed energy to the scintillant emits light.
Liquid scintillation counting is the main challenge to applications of organic scintillators in the field of nuclear medicine. Here, the scintillator is liquid and is dissolved with the radioactive sample to be assayed. The activity of low energy β-emitting radionuclides like 14C and 3H can be measured using this method.
Gaseous Scintillators
The concept of using liquefied noble gases as scintillation material was developed around 60 years ago. The properties of condensed noble gas scintillators are reviewed in the following section.
High-pressure noble gas scintillators
The key benefits of using gas scintillation detectors:
- The size and shape of the scintillation volume are flexible
- Fast scintillation decay times
- 2π and 4π geometry are easy to obtain
- The stopping power obtained due to the variation in gas pressure can be controlled, so that the conditions can be adjusted. Thus the energy is completely dissipated by the charged particles in gas
- Linearity of the scintillation response can be obtained over a wide range of E and dE/dx
Gases have to be used at high pressures to improve the detection efficiency. Proportional counters use a gas pressure of less than 2 MPa as providing high voltages is difficult.
The advantages of using gas scintillators:
- Avoids the need for high voltage bias
- It can withstand high pressure
- Fast scintillation signals can be used for the measurement of nanosecond time range
- It can suppress the emission of gamma rays
Other commonly used gas scintillators include:
Single-Channel Gas Scintillators
A typical example of this type of gas scintillator is a high pressure 3He scintillation neutron detector. This detector consists of a high-pressure chamber and aluminum reflectors. The reflectors are coated with VUV high-reflectance paint and p-terphenyl wavelength shifter coating, which is optically thick. Internal window surfaces are also coated with p-terphenyl and metal gaskets are assembled in the detector. Mu-metal is coated inside the chamber for reducing external magnetic interferences.
Multi-Channel Gas Scintillators
A two-channel, high pressure 3He scintillation detector consists of two photodetectors within a stainless steel shell and silicon avalanche photodiodes whose surface is deposited with a p-terphenyl wave shifter. Photodiodes transmit scintillation signals with correlated values in coincidence operation mode of the detector.
Sources and Further Reading
- Scintillators as Gamma-ray Detectors: http://imagine.gsfc.nasa.gov/docs/science/how_l2/gamma_scintillators.html
- Tsoulfanidis. N, Andsberger. S, 2011, Measurement and Detection of Radiation, Boca Raton, FL,Taylor & Francis Group.
- McParland.B.J, 2010, Nuclear Medicine Radiation Dosimetry: Advanced Theoretical Principles, London, Springer Dordrecht Heidelberg.
- Aprile E, Bolotnikov A.E, Bolozdynya J.I, Doke. T, 2006, Noble Gas Detectors, Weinheim, WILEY-VCH Verlag GmbH & Co.
This article was updated on 17th February, 2020.