Gas sensors, especially carbon dioxide sensors, are becoming increasingly important in environmental monitoring, as well as seeing more use in safety monitoring for industrial processes. As a result, demand for robust, low-cost gas sensors is rising.
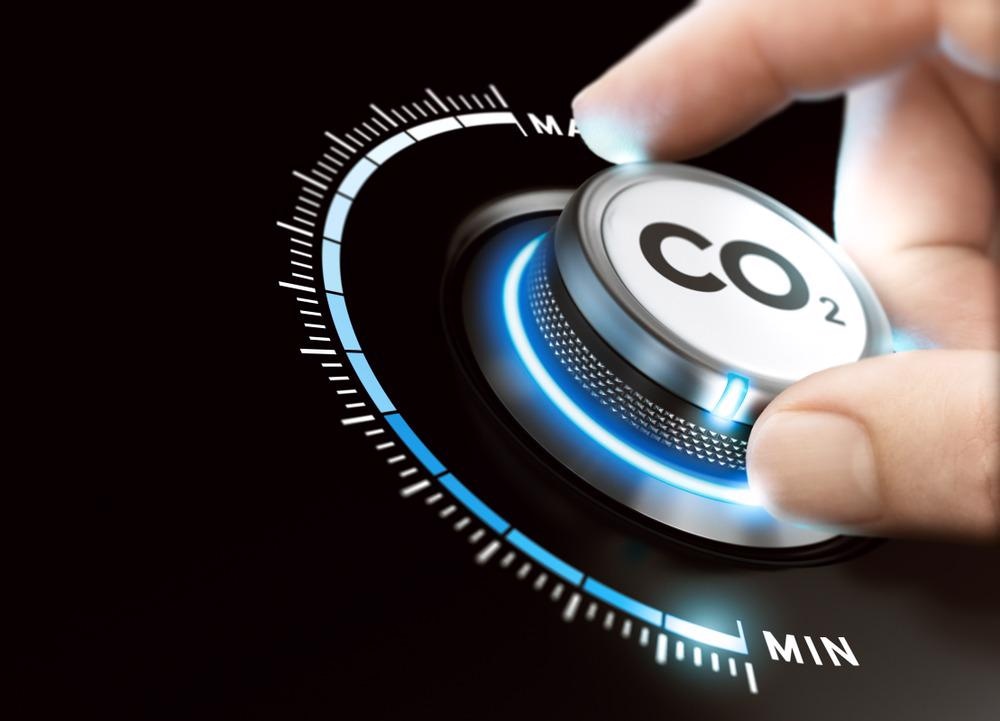
Image Credit: Olivier Le Moal/Shutterstock.com
In a recent study, University of Cambridge engineers presented a miniaturized thermal acoustic gas sensor made with a CMOS microhotplate and MEMS microphone, arguing that their novel gas sensor could meet this rising need for gas sensors.
How Do Carbon Dioxide (CO2) Gas Sensors Work?
Gas sensing is increasingly important. In particular, carbon dioxide (CO2) detection offers insight into both indoor air quality and external conditions.
CO2 is an asphyxiant gas that can cause suffocation and unconsciousness within a few minutes when exposed to humans in concentrations greater than 70,000 ppm (7%.) Gas sensors are used to detect hazardous levels of atmospheric pollutants, including CO2, nitrogen dioxide (NO2,), and volatile hydrocarbons like benzene (C6H6.)
There is no universal approach to gas sensing at present, but most common gas sensors utilize various transduction effects such as gas-induced changes in the electrical, optical, physical, and thermal qualities of materials.
Chemiresistors manipulate changes in the electrical conductivity of a layer of semiconducting metal oxide (MOx) that is exposed to an oxidizing or reducing gas. Chemiresistors are used in low-cost, low-power applications such as portable air conditioning (A/C) units. Chemiresistors are highly sensitive to organic compounds, but have poor selectivity and cannot achieve CO2 detection.
Optical sensors detect absorption lines in the mid-infrared (MIR) spectrum. This type, which includes photoacoustic sensors, does address some of the limitations that chemiresistors face.
As optical sensors are capable of measuring carbon dioxide, they are the primary type currently used for CO2 detection, as well as other gas sensing systems. However, applications for optical sensors are currently limited by relatively high manufacturing costs, especially for the micromachining processing of chip-scale devices.
Cheaper alternative gas sensing technologies have recently been experimentally reported. These were based on CMOS thermal conductivity sensors that can exploit differences in thermal conductivity between different gasses.
A New Gas Sensor for CO2 Detection
A team of engineers from the University of Cambridge, UK, and Flusso Ltd (a Cambridge spin-out company established in 2016 to commercialize innovative sensor technology) recently presented a novel gas sensor design and experimental results achieved with the device in the journal Scientific Reports.
The CO2 gas sensor was made using a complementary metal–oxide–semiconductor (CMOS) microhotplate and a microelectromechanical systems (MEMS) microphone, and works as a thermal acoustic gas sensor. The CO2 gas sensor detects changes in the thermal acoustic conversion efficiency, which depends on the physical properties of gas. It combines an active sensing element (the MEMS microphone) with a reference to compensate for acoustic noise.
Thermal acoustic systems create acoustic waves in gas and have been used previously as transducers and components for converting thermal acoustic power into electrical energy. Until now, however, there had been little work done to apply the technology to gas sensing.
CMOS is a miniaturized fabrication process that uses metal-oxide-semiconductor field-effect transistors (MOSFETs) for logic functions. It is commonly used to make integrated circuit (IC) chips such as microprocesses and memory chips. CMOS devices typically have high noise immunity and low static power consumption, making them well-suited for passive sensing applications.
MEMSs are microscopic devices with moving parts. MEMS devices are generally sized between 20 μm and 1,000 μm (0.02 mm to 1.0 mm), and their components are between 1 μm and 100 μm in size (or 0.001 mm to 0.1 mm). The final chip’s dimensions were just 1.08 mm × 1.08 mm × 0.38 mm.
The new CO2 sensor does not require gas-encapsulated microphones or optical filters, unlike other photoacoustic gas sensors currently available. It also benefits from CMOS technology: production is scalable, the device is low cost, and it can be effectively miniaturized.
The authors of the paper say the CO2 gas sensor could be used for gas leak detection in an industrial plant, for example.
The CO2 gas sensor has a circular membrane made out of silicon dioxide (SiO2) measuring 4.6 μm thick and 600 μm in diameter. A 300 μm diameter tungsten (W) microheater was embedded in the SiO2 membrane. W has a very high melting point (3,400 °C) and low susceptibility to electromigration. This made for stable electro-thermal performance in the sensor.
The heater is thermally isolated from the silicon (Si) substrate material for the device by the membrane, which was formed using the deep reactive ion etching (DRIE) technique.
The CO2 gas sensor is constructed by mounting the microheater chip above the port of an analog commercial off the shelf MEMS microphone. The configuration is simple, miniature (non-resonant.)
The engineers used die mounting pads to mechanically secure the microheater chip to the microphone substrate, forming an acoustic cavity between the chip’s etched membrane and the microphone port. A small opening (around 500 μm × 50 μm) between the die mounting pads allowed gas to diffuse into the cavity.
At room temperature, the device’s heater track had electrical resistance of 39 Ω. A p+–n+ junction thermal diode imbedded in the SiO2 membrane enables the microheater chip to monitor its own operating temperature.
References and Further Reading
Hopper, R., D. Popa, F. Udrea, et al (2022). Miniaturized thermal acoustic gas sensor based on a CMOS microhotplate and MEMS microphone. Scientific Reports. Available at: https://doi.org/10.1038/s41598-022-05613-0.
Nazemi, H., A. Joseph, J. Park, et al (2019). Advanced Micro- and Nano-Gas Sensor Technology: A Review. Sensors. Available at: https://doi.org/10.3390/s19061285.
Disclaimer: The views expressed here are those of the author expressed in their private capacity and do not necessarily represent the views of AZoM.com Limited T/A AZoNetwork the owner and operator of this website. This disclaimer forms part of the Terms and conditions of use of this website.