Energy harvesting is moving fast, and at the heart of that progress are some seriously advanced materials. These next-generation materials are making it easier and more efficient to convert ambient energy into usable power. We're talking about materials that can help turn body movements, heat, or even the touch of a fingertip into electricity.1-4
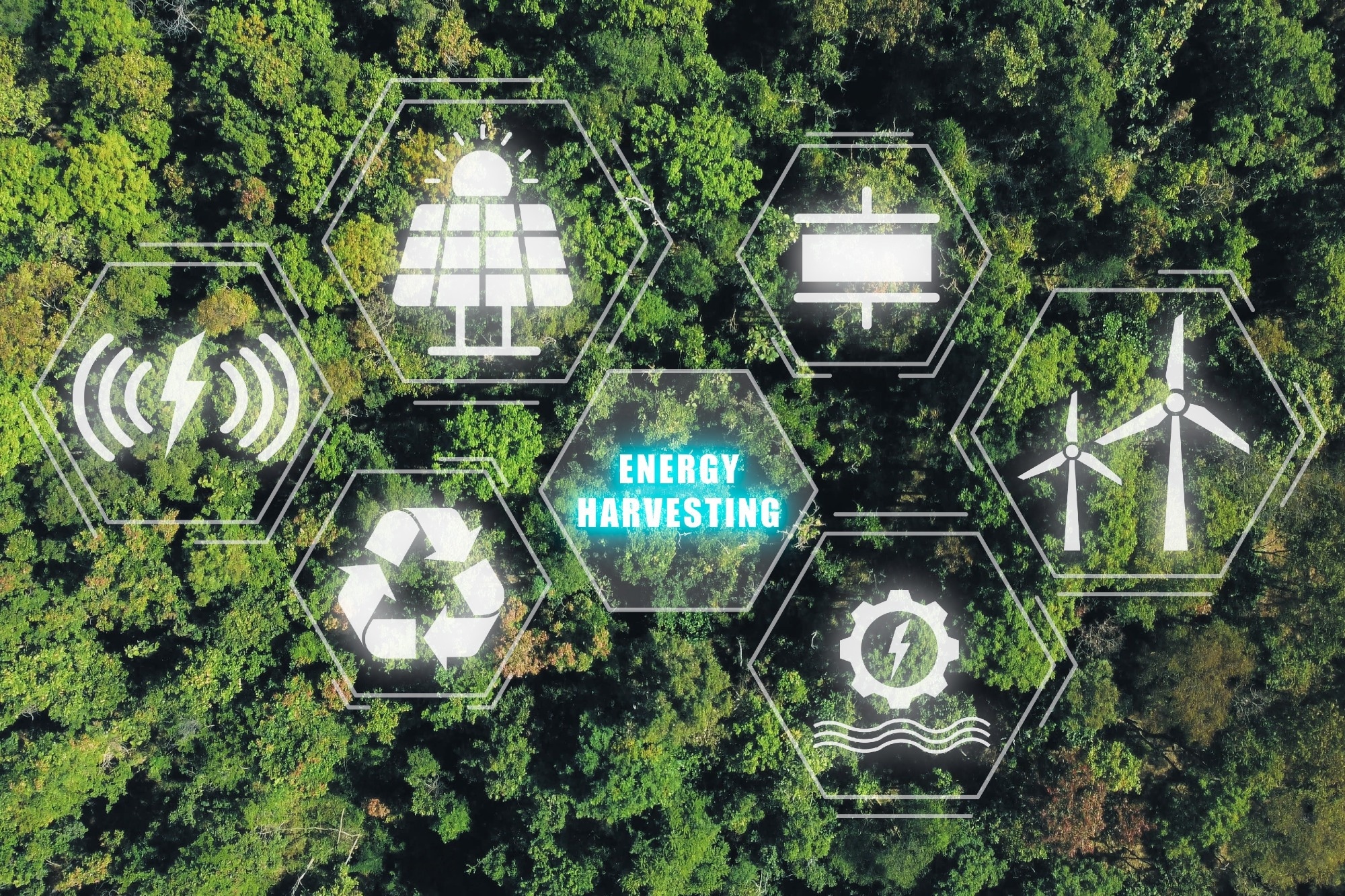
Image Credit: Mongta Studio/Shutterstock.com
Let’s take a look at what’s driving this shift and why it matters.
Download your PDF copy now!
Why New Materials Are a Big Deal
Energy harvesting isn’t exactly new. We’ve had solar panels and thermoelectric devices around for years. But what’s changing is the range of materials available—and how good they’ve gotten at pulling energy from everyday surroundings.
Instead of relying solely on traditional batteries, small and mobile electronics can now tap into the environment around them. We're seeing smart ways to capture energy from sunlight, body heat, movement, even pressure and magnetic fields. And this leap forward is largely thanks to materials science, especially when it comes to micro- and nanoscale materials that make these systems smaller, smarter, and more efficient.1,2
Triboelectric Materials
Kicking things off, triboelectric materials take advantage of something you’ve probably experienced firsthand: static electricity. When two different materials rub together, they exchange charges. Triboelectric nanogenerators (TENGs) use this effect to turn mechanical motion, like tapping, sliding, or pressing, into electrical energy.
These materials are simple, cost-effective, and easily customized. They've already shown promise in pressure-sensitive sensors, especially when layered into thin, flexible films. One standout example features nanowires on a triboelectric layer that dramatically improve charge generation, enabling highly responsive, self-powered touch sensors.
And this isn’t just about touchscreens or smart surfaces. TENGs can also convert larger motions—like rotation or displacement—into usable electricity. That leads us right into another class of materials doing similar work, but in a slightly different way.1,2
What is the triboelectric effect? | ACCIONA #Imnovation
Piezoelectric Materials
While triboelectrics harness surface friction, piezoelectric materials dive deeper, generating electricity when compressed or stretched. These materials respond to mechanical stress at a structural level, making them ideal for harvesting energy from motion, pressure, and vibration.1-4
Recent developments have made piezoelectric systems thinner, more flexible, and surprisingly powerful. We're talking about ceramics that can be deposited at low temperatures, or films with built-in stress that double their energy output. Some devices now use them to generate power from things like shoe impacts, engine vibrations, or even the beating of a heart.
Lead-free options like potassium sodium niobate (KNN) are especially important in medical applications. Their safety and performance make them great candidates for implants or wearable health monitors. And with the growing demand for bio-integrated tech, that’s an area seeing major momentum.
The transition from mechanical to thermal energy sources brings us to the next set of materials—ones that turn heat, rather than movement, into power.
Thermoelectric Materials
Where piezo materials respond to motion, thermoelectric materials are all about heat, specifically, differences in temperature. These materials convert a heat gradient directly into electricity using the Seebeck effect, making them perfect for situations where waste heat is plentiful: engines, electronics, or even body heat.
What makes today’s thermoelectrics stand out is the balance of electrical performance and thermal control. Materials like skutterudites are especially efficient, combining good conductivity with low thermal losses. And in solar thermoelectric generators (STEGs), heat from sunlight can be turned into power using passive systems with no moving parts.
These technologies are increasingly being adapted for home use, not just space missions or industrial applications. And hybrid designs are pushing the envelope even further. Some systems now pair solar collectors with thermosyphons to achieve up to 50 % efficiency under lab conditions—a big leap for solid-state power generation.
Of course, heat doesn’t always come in large, consistent amounts. Sometimes, it comes in quick bursts or subtle changes. And that’s where pyroelectric materials come into play.1,2
Pyroelectric Materials
Unlike thermoelectrics, which need a steady temperature difference, pyroelectric materials react to changes in temperature. When heated or cooled suddenly, they generate an electric charge, making them ideal for intermittent thermal environments or systems that cycle on and off.
One exciting area of development here is nanowires. Zinc oxide nanowire arrays, for example, have outperformed traditional thin films, producing higher voltages in smaller packages. These materials can even charge batteries or power simple displays with a single pulse of heat.
Researchers are also experimenting with ways to tune the energy output. In some cases, flexible nanogenerators made from lead-free materials can be modified by changing electric fields, which shift how their internal domains align. Other designs use etched electrodes or mesh layers to improve energy collection, boosting overall efficiency with clever geometry.1,2
2D Materials
One of the most exciting developments in energy harvesting lately has come from materials that are almost impossibly thin. We're talking just a few atoms thick—welcome to the world of two-dimensional (2D) materials.
Graphene, molybdenum disulfide (MoS2), and black phosphorus are among the standout players. These atomically thin materials combine excellent electrical conductivity, mechanical flexibility, and tunable electronic properties, making them ideal for building smaller, smarter, and more adaptable energy harvesters.
Thanks to their flexibility and sensitivity, 2D materials are already enabling devices that move with the human body while generating power from even the tiniest motions. Think of a graphene-based piezoelectric nanogenerator that stretches like skin but still produces usable electricity. These materials are also being integrated into ultra-thin solar cells and thermoelectric devices, pushing performance and miniaturization to new levels.
As research deepens, expect 2D materials to take center stage in next-gen wearables, soft robotics, and even self-powered medical implants.
But as we make devices thinner and more flexible, we also have to make them tougher, which brings us to another critical piece of the puzzle...
Self-Healing and Durable Materials
Durability is a major concern for energy harvesters, especially in wearables or devices exposed to stress, moisture, or everyday wear and tear. That’s where self-healing materials come in—smart polymers and composites that can repair themselves after damage, much like biological tissue.
Imagine your fitness tracker bouncing back after a scratch or your wearable sensor recovering from repeated flexing. Some of the latest triboelectric and piezoelectric devices are being built from self-healing elastomers that automatically restore their structure and electrical functionality when damaged.
This isn’t just a cool trick; it’s a big step toward making energy harvesting systems more resilient and longer-lasting. Especially in scenarios like medical implants or remote sensors, where repair isn't practical, self-healing capabilities could be the difference between a device that lasts months and one that works for years.
And speaking of getting more from your devices, why stop at harvesting one kind of energy when you can tap into several at once?
Hybrid and Multifunctional Materials
Hybrid energy harvesting systems are quickly becoming a go-to solution for maximizing power output. These materials combine multiple mechanisms—like triboelectric, piezoelectric, and photovoltaic effects—into a single device, enabling them to pull energy from different sources all at once.
Picture a single patch that harvests energy from footsteps via a piezoelectric layer, sunlight through a thin-film solar cell, and then stores it all in an integrated supercapacitor. It’s not just about generating more energy—it’s about creating more consistent and reliable power for small electronics, wearables, and IoT devices.
These multifunctional systems are already being explored for use in smart clothing, self-sustaining sensors, and autonomous wireless networks that could operate for years without ever needing a battery swap. It’s energy harvesting that works smarter, not harder.
Of course, pushing boundaries often means exploring completely new classes of materials—and there’s a fresh wave of those coming too.
Emerging Materials: MXenes, Perovskites, and Beyond
Beyond the more established materials, a new generation is emerging with even more ambitious potential. MXenes—2D materials made from transition metal carbides and nitrides—stand out for their high conductivity, tunable surface properties, and potential in hybrid energy harvesting and storage devices.
Then there are perovskites. While they’re best known for their role in high-efficiency solar cells, they’re now gaining attention for their piezoelectric and thermoelectric capabilities as well. Scientists are learning how to tweak their structure at the molecular level for flexible, transparent, and highly efficient energy devices.
And it doesn’t stop there. Organic-inorganic hybrids, biodegradable composites, and eco-friendly alternatives are also making waves, all with an eye toward higher performance, better scalability, and more sustainable manufacturing.
Still, even with all this innovation, bringing these materials out of the lab and into everyday products isn’t always straightforward.
Integration and Real-World Challenges
As promising as all these materials are, translating lab success into real-world performance is no small feat. One major hurdle is scalability—how do we make these materials in large volumes without skyrocketing costs? Another is integration: getting them to work seamlessly with existing electronics, packaging, and systems.
There's also the issue of reliability. Devices have to perform not just in ideal conditions but in messy, unpredictable real-life environments. That means dealing with humidity, wear and tear, long-term stability, and power consistency.
To move forward, the field needs clear performance benchmarks—standards that make it easier to compare materials, refine designs, and tailor devices to specific needs. Power density, energy conversion efficiency, and durability all need to be measured and optimized across the board.
Once those pieces are in place, the path becomes much clearer, and the potential applications become even more exciting.
Conclusion
What we’re seeing across energy harvesting research isn’t just a collection of new materials; it’s a shift in how we think about power. Each innovation, whether it's a 2D layer just atoms thick or a self-healing polymer that bounces back from damage, brings us closer to devices that can operate longer, adapt better, and rely less on traditional batteries.
These materials aren’t competing, they’re converging. Flexible sensors powered by motion, solar cells that double as heat harvesters, systems that charge from multiple energy sources at once: it’s all part of a broader move toward integrated, intelligent power solutions that work with the world around them, not against it.
The path from lab to product is still full of challenges—scalability, reliability, and seamless integration remain major hurdles. But the direction is clear. Energy harvesting is no longer just about capturing stray power, it’s about designing systems that make energy use more local, efficient, and responsive.
As research and real-world applications continue to align, the next generation of electronics won’t just be smart. They’ll be self-sufficient.
Want to Explore What’s Next?
Check out innovations in stretchable batteries, biodegradable sensors, or AI-powered energy management systems. The future of energy harvesting isn’t just about capturing power—it’s about rethinking how we interact with it, everywhere we go.
Download your PDF copy now!
References and Further Reading
- Tong, C. (2018). Emerging materials for energy harvesting. Introduction to Materials for Advanced Energy Systems, 719-817. DOI: 10.1007/978-3-319-98002-7_11, https://link.springer.com/chapter/10.1007/978-3-319-98002-7_11
- Mehta, S., Abougreen, A. N., & Gupta, S. K. (2024). Emerging Materials, Technologies, and Solutions for Energy Harvesting. IGI Global. DOI:10.4018/979-8-3693-2003-7, https://www.researchgate.net/publication/378943572_Emerging_Materials_Technologies_and_Solutions_for_Energy_Harvesting
- Banerjee, S., Bairagi, S., & Ali, S. W. (2021). A critical review on lead-free hybrid materials for next-generation piezoelectric energy harvesting and conversion. Ceramics International, 47(12), 16402-16421. DOI: 10.1016/j.ceramint.2021.03.054, https://www.sciencedirect.com/science/article/abs/pii/S0272884221007185
- Li, T., Lee, P. S. (2022). Piezoelectric energy harvesting technology: from materials, structures, to applications. Small Structures, 3(3), 2100128. DOI: 10.1002/sstr.202100128, https://onlinelibrary.wiley.com/doi/full/10.1002/sstr.202100128
Disclaimer: The views expressed here are those of the author expressed in their private capacity and do not necessarily represent the views of AZoM.com Limited T/A AZoNetwork the owner and operator of this website. This disclaimer forms part of the Terms and conditions of use of this website.